[[Waves, Tides, Tidal Nodes, and Amphidromic Points
]][Tides intro by shane](https://publish.obsidian.md/Attachments/Tides+intro+by+shane+1+1+1+1+1.mp4)
[Tide Dynamics](https://publish.obsidian.md/Attachments/Tide+Dynamics+2+1+1+1+1.pdf)
[https://publish.obsidian.md/shanesdatabase](https://publish.obsidian.md/shanesdatabase)
Title
Contents
[https://www.seafriends.org.nz/oceano/tides.htm](https://www.seafriends.org.nz/oceano/tides.htm)
.gif)
Moon phase is biggest contributor to tides on earth, what is mainstream explanation for this?
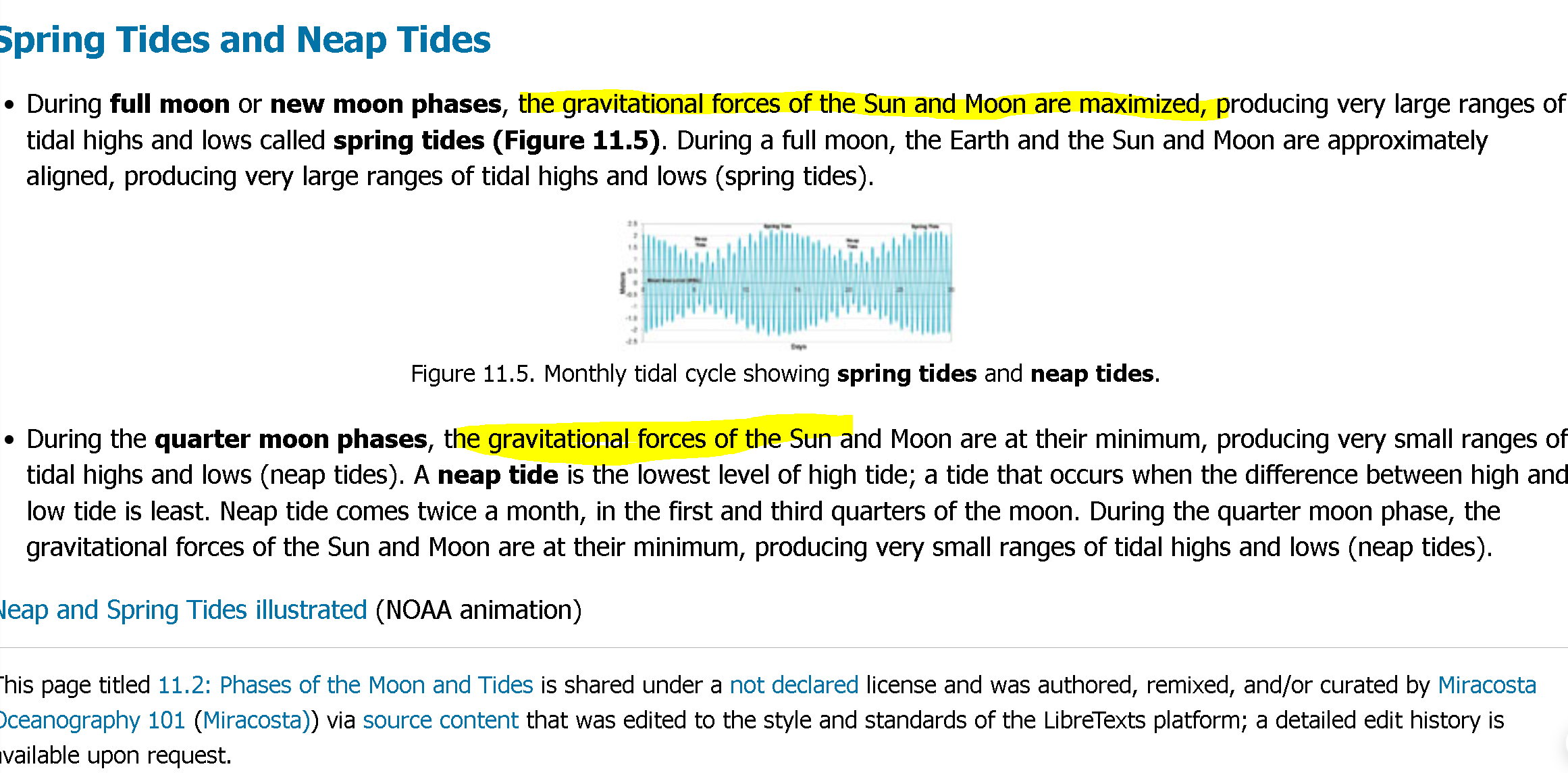
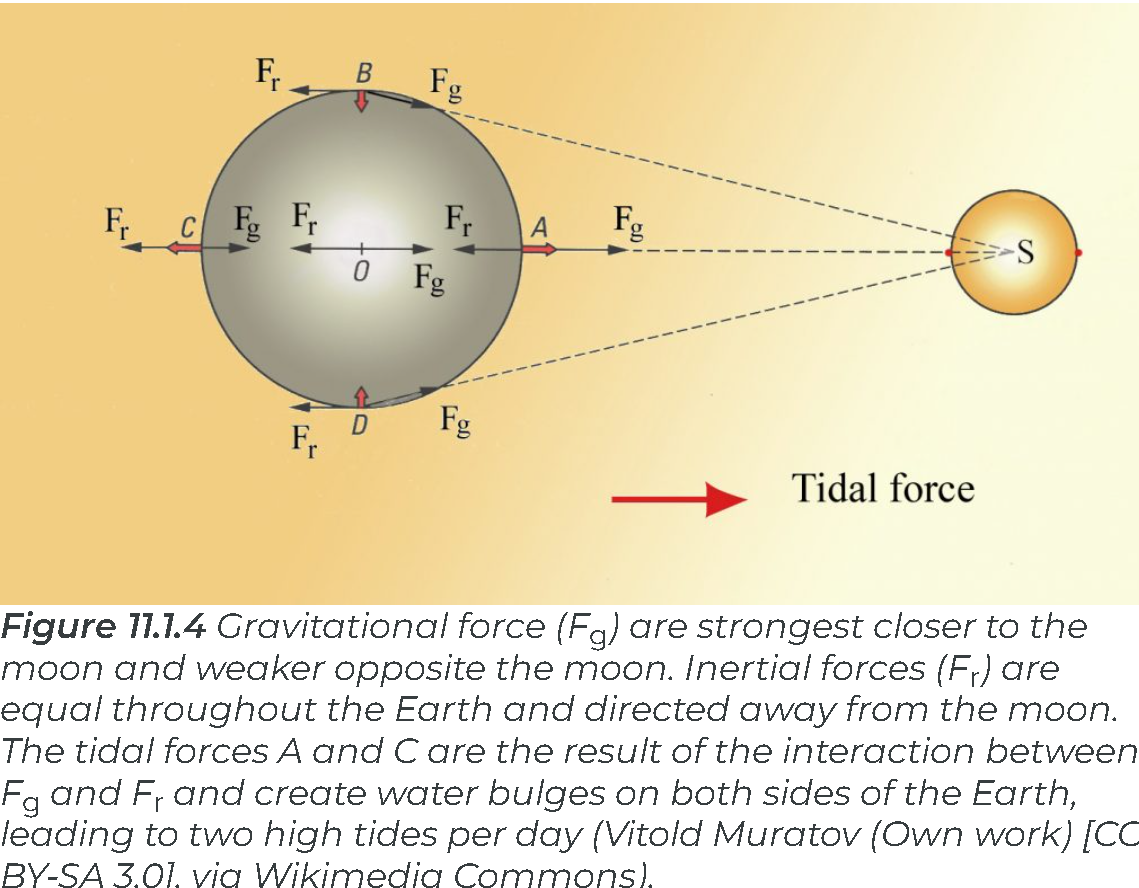
When the sun, Earth and moon are at 90o to each other, the solar and lunar bulges are out of phase, and cancel each other out ([destructive interference](https://rwu.pressbooks.pub/webboceanography/chapter/11-1-tidal-forces/#term_334_831)). Now the tidal range is small, with low high tides and high low tides (Figure 11.1.6). These are **[neap tides](https://rwu.pressbooks.pub/webboceanography/chapter/11-1-tidal-forces/#term_334_1117)**, and occur every two weeks, when the moon is in its 1/4 and 3/4 phases (Figure 11.1.7).
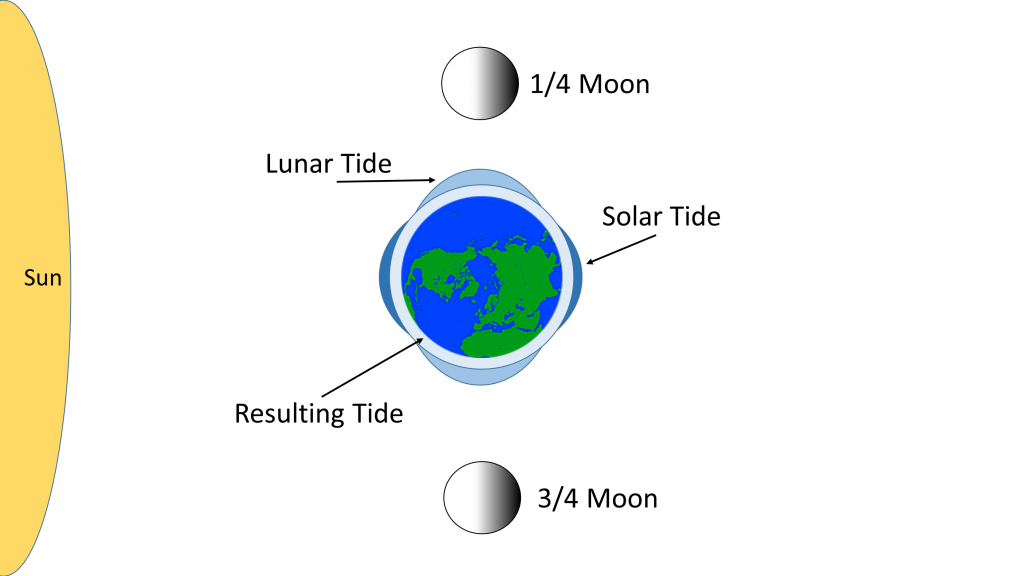
**Figure 11.1.6** Neap tides are created during 1/4 and 3/4 moons when the Earth, sun and moon are perpendicular to each other. The solar and lunar tides cancel each other out, resulting in a small tidal range (PW).
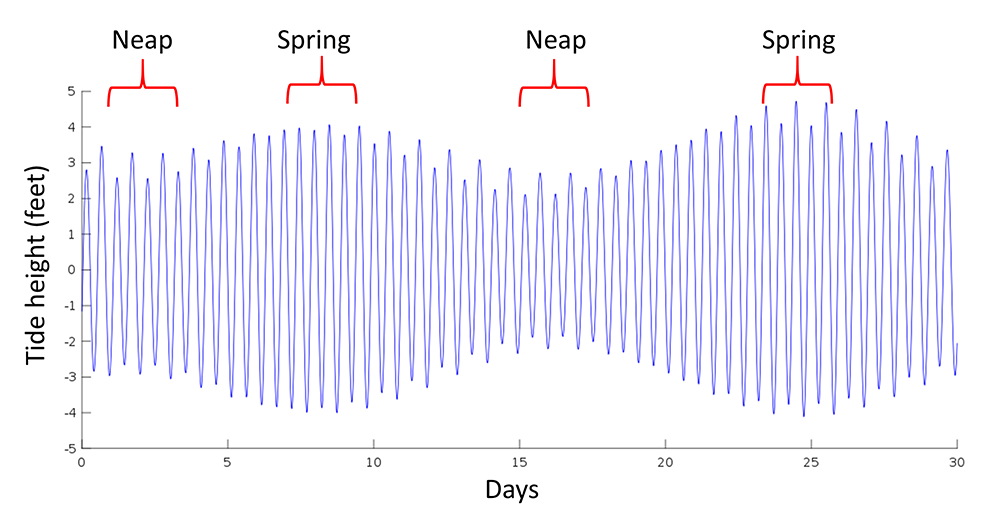
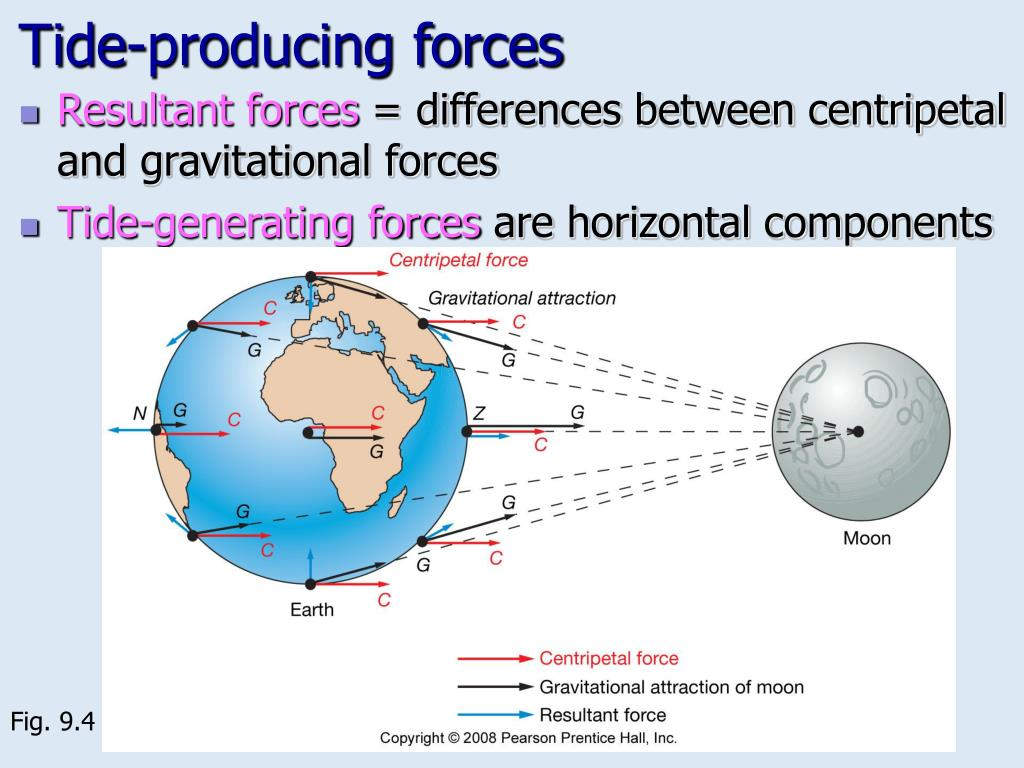

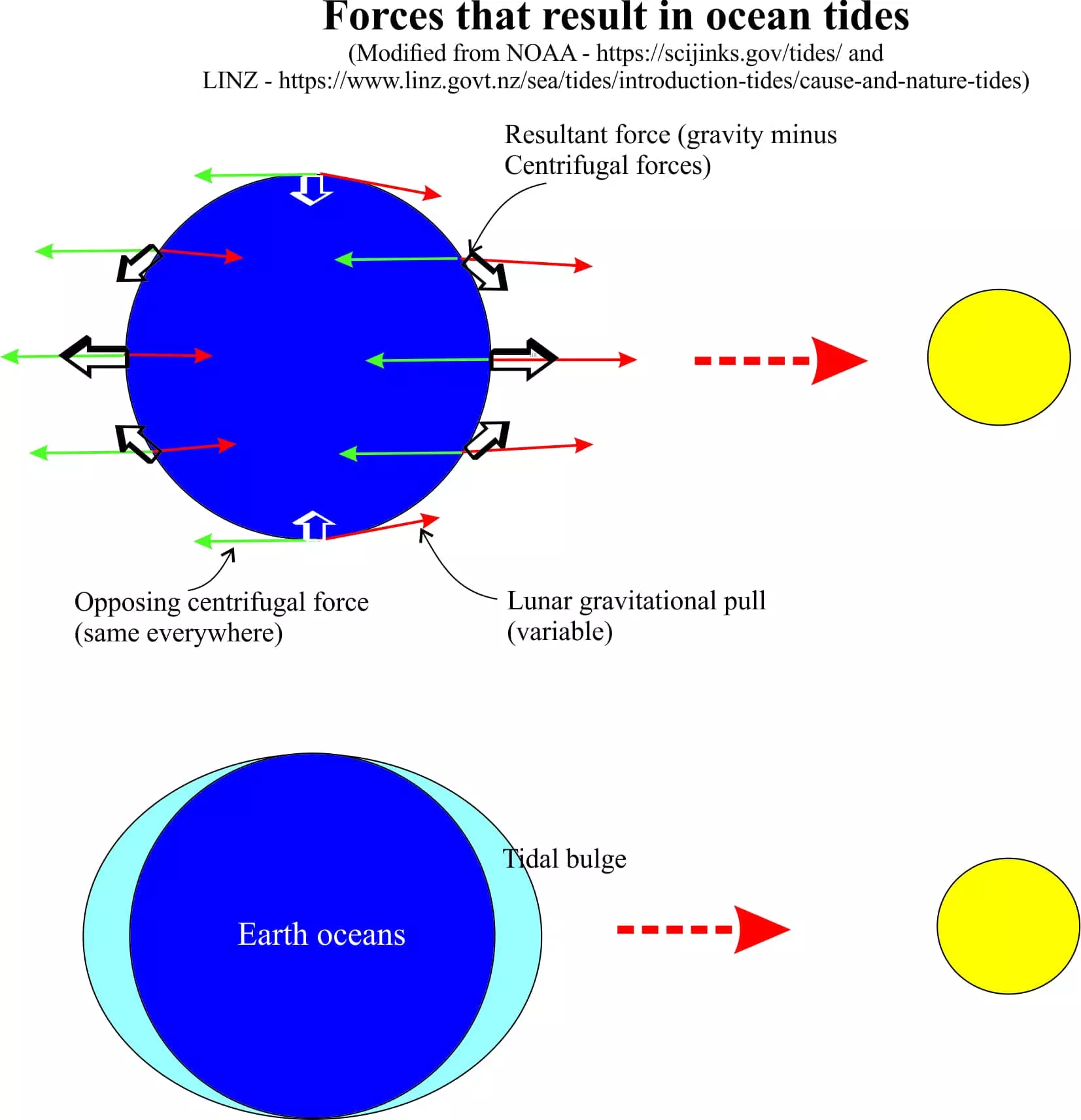
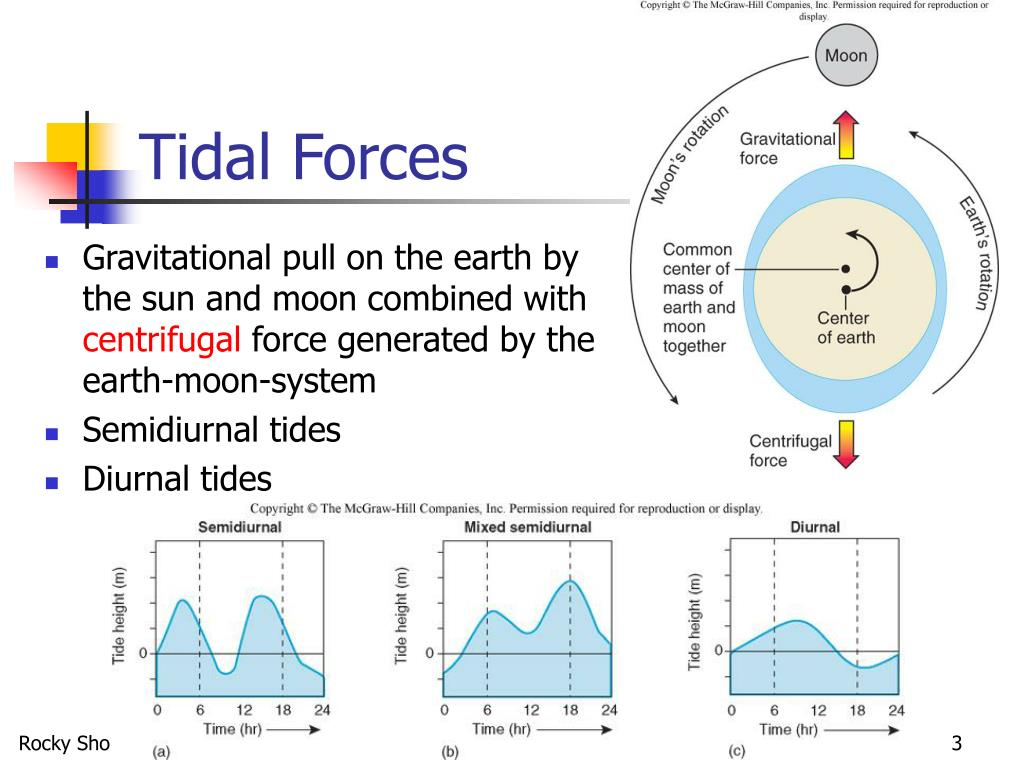
Tidal Causes
[https://www.youtube.com/watch?v=eMX--80J_5o&ab_channel=EarthRocks%21](https://www.youtube.com/watch?v=eMX--80J_5o&ab_channel=EarthRocks%21)
[00:00:03]
First of all, we know that most tidal waves have a period of one wave every 12 hours and 25 minutes, otherwise stated as two waves every 24 hours and 50 minutes. What else has that same period? Earth's rotation relative to the moon is also 24 hours and 50 minutes. What does that mean? After the Earth is rotated once relative to the sun 24 hours, it has to rotate another 50 minutes to catch up with the moon, which during that 24 hours moved 1/29 its way around its orbit around the Earth.
24 hours and 50 minutes is a lunar day. In addition, the two week cycle of neap and spring tides exactly matches the phases of the moon as the moon completes its orbit around the Earth. The moon is the main agent causing tides on our planet. How? This image, which is not to scale, shows a spherical blue earth that is being pulled to the right by a gravitational force between itself and the moon. The side of the Earth closest to the moon has a longer arrow, representing the stronger gravitational force felt there. The arrow on the opposite side is shorter because its force is smaller.
Gravitational force is stronger the closer two objects are together and weaker the further away they are. Why? The gravitational force experienced between any two objects is equal to a very small constant number g, known as the gravitational constant, multiplied by the masses of both objects and divided by the distance between them squared. How would you make this gravitational force or fraction greater? Increase the numerator or decrease the denominator. Increase the mass of the objects or decrease the distance between the two objects. The force of gravity on the closer side to the moon thus feels a stronger gravitational force than the other. If we take the medium sized gravitational force between Earth and Moon experienced at the center of the Earth, and subtract it from all the other force arrows found around the planet, we get this image, which shows the net tidal forces experienced on all points of Earth's surface as a result of varying gravitational forces, strength and direction.
What you can see from the direction of the arrows is that water on Earth's surface would be pushed away from these areas at right angles to the moon, and towards these areas that are in line with the moon.
This movement of water acts as a global squeezing into two bulges, one facing the moon, the other on the opposite side of the moon, and the solid Earth rotates under these bulges, carrying various parts of the planet into and out of these bulges throughout the day. When you're standing under a bulge, you are experiencing a high tide. When you are between two bulges, you experience a low tide. The water has been pulled away. How does the sun fit in? The moon is pulling the ocean to create a tidal bulge when it aligns with the centrifugal force, which itself is also oscillating around the earth. The moon's tide generating force is twice as important as the sun. The gravitational force that's felt between the Earth and the sun is greater than that felt between the Earth and the moon, but it isn't the total force that creates the tides. It's the difference in force felt on one side of the Earth versus the other. And the difference becomes greater the closer the two objects are to each other.
This graph shows how force of gravity increases with proximity and decreases with distance. The sun is so massive its force is much greater than the moon's, even though it's further away. But again, it's the difference in force that creates tides. So let's look at what happens when you move the moon or any object further away. For the close moon, when we take the difference between the near side force and the far side force, we get a force differential this large. When we move the moon further away, we see that the near side force and far side forces vary less. The force differential is smaller, so the tides would be smaller. This cartoon says the same thing in a much more clever way.
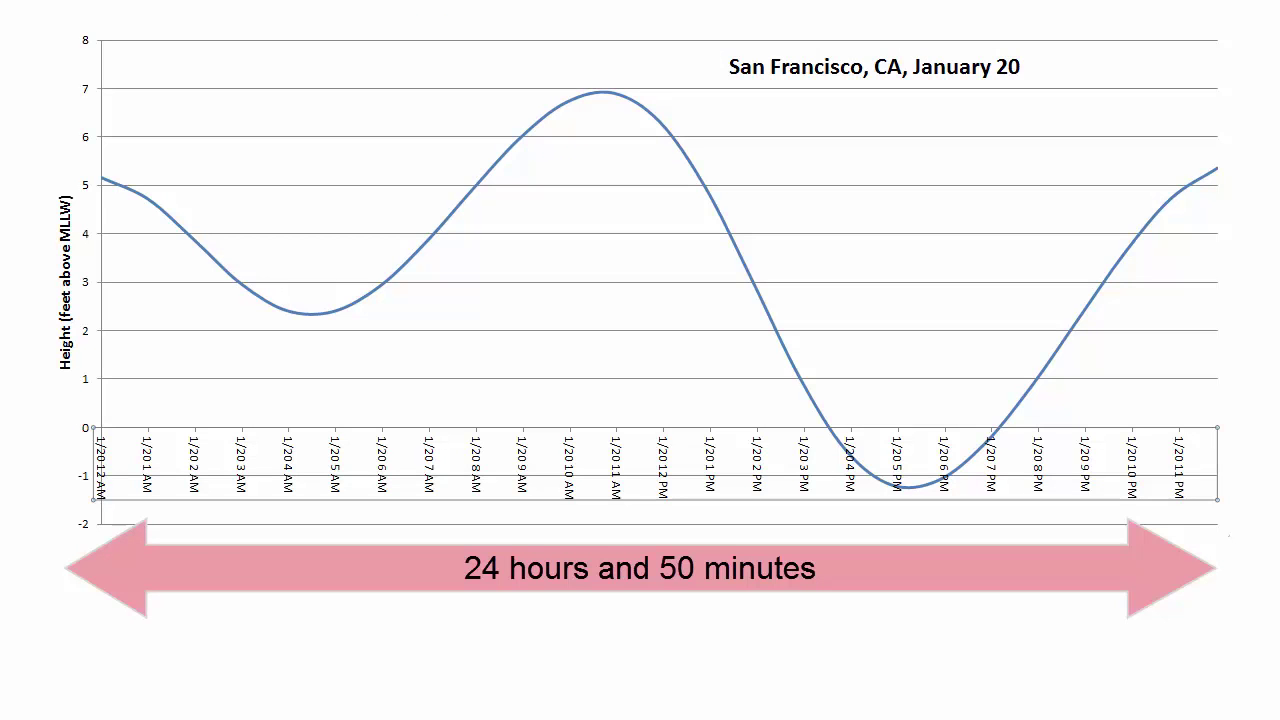
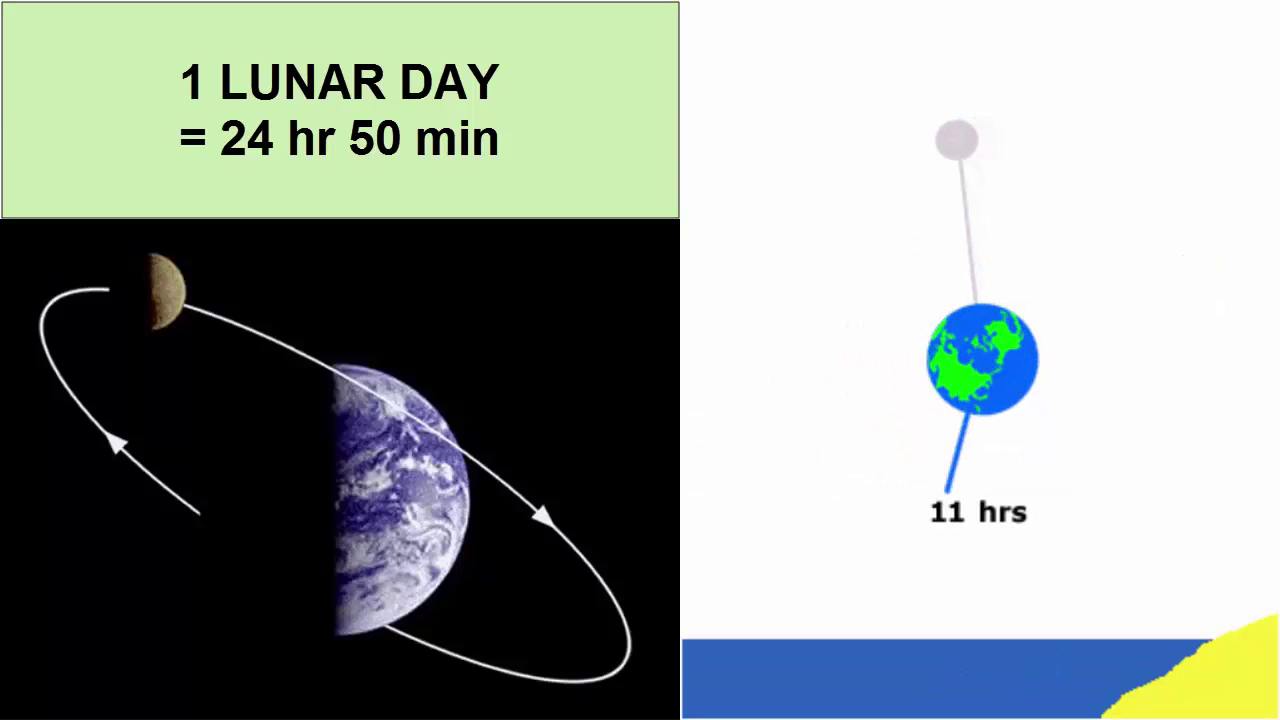
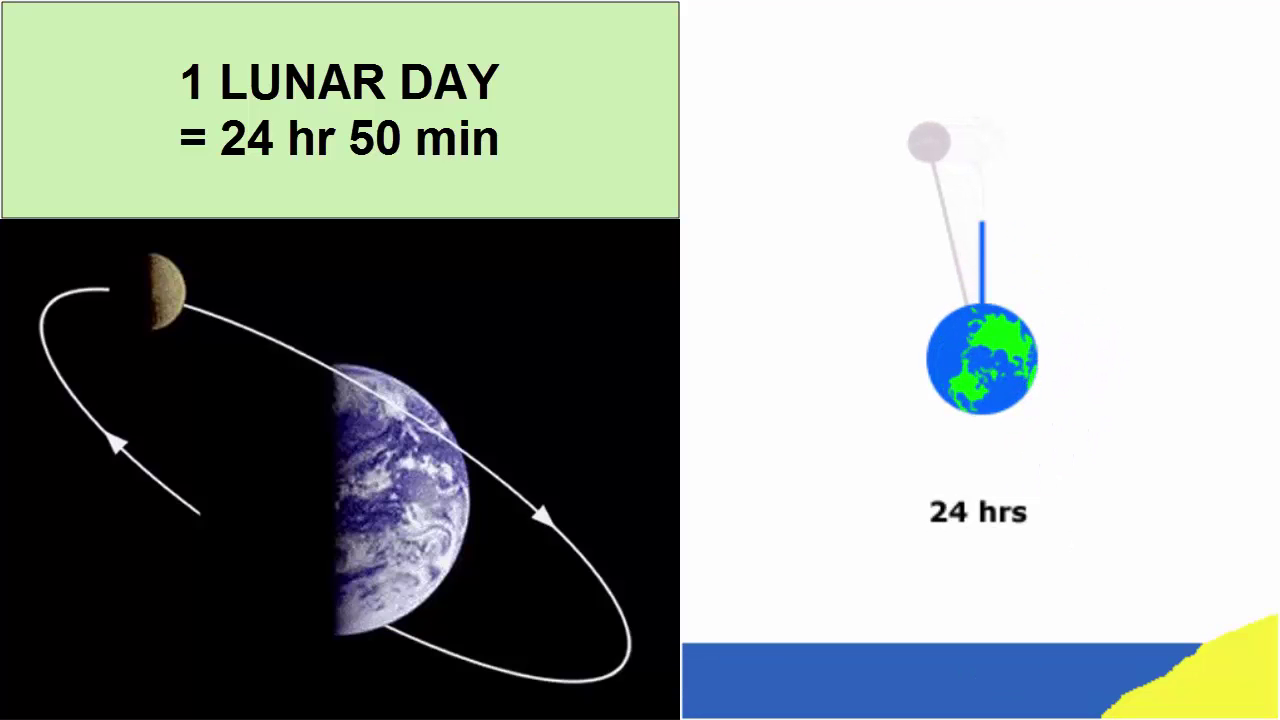

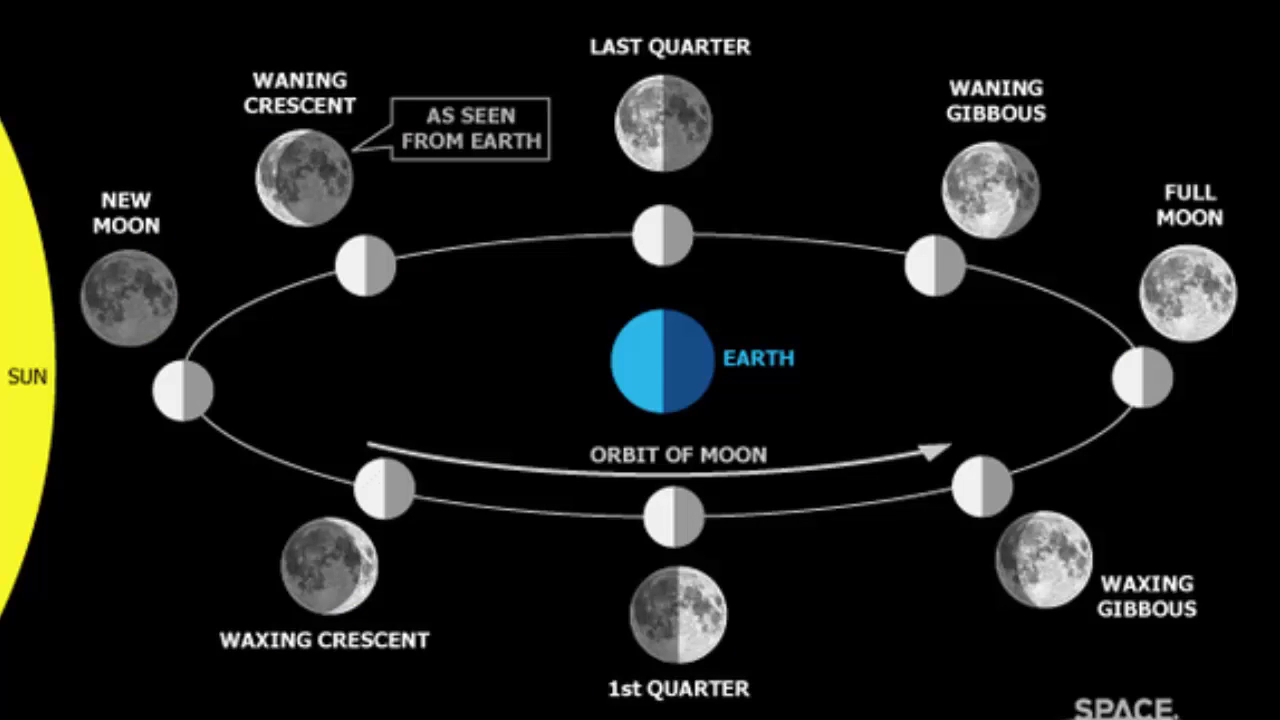
[00:04:26]
The sun is a much more massive body than the moon. However, because it is so far away from the Earth, the differences experienced on the near and far sides are much, much smaller than those produced by the moon. We can show that more clearly in this image, which is also not to scale, but nonetheless shows that there are always two bulges associated with the solar tides one on the side facing the sun, which is being pulled toward the sun with a greater force than the other on the other side, away from the sun, which is also being pulled toward the sun. But as it's further away, not as strongly. The resulting force differentials cause again, the water to get pushed away from the points on the surface at right angles to the sun, and push toward the areas that are in line with the sun. The lunar bulges are situated similarly with respect to the moon. Notice the difference in force, shown as differences in arrow length are much greater for the lunar bulges than for the solar bulges, and hence the lunar tides are the dominant ones and the ones that dictate tidal period end patterns
The lunar and solar bulges are continually interfering with each other. As the moon moves around the Earth every 29 days, it will carry the lunar bulges into phase and out of phase with the solar bulges. When the two are in phase, which happens during full and new Moon's, the two bulges constructively interfere and we experience a much greater tidal range with lower lows and higher highs. Spring tides. When the two bulges are out of phase, which happens when we see half moons, the interference is destructive and the tidal range is at its lowest. The lows aren't so low. The highs aren't so high. Neap tides. Turns out we can walk outside at night, look up into the night sky and based on the phase of the moon, determine whether we're experiencing neap or spring tides. Pause now.
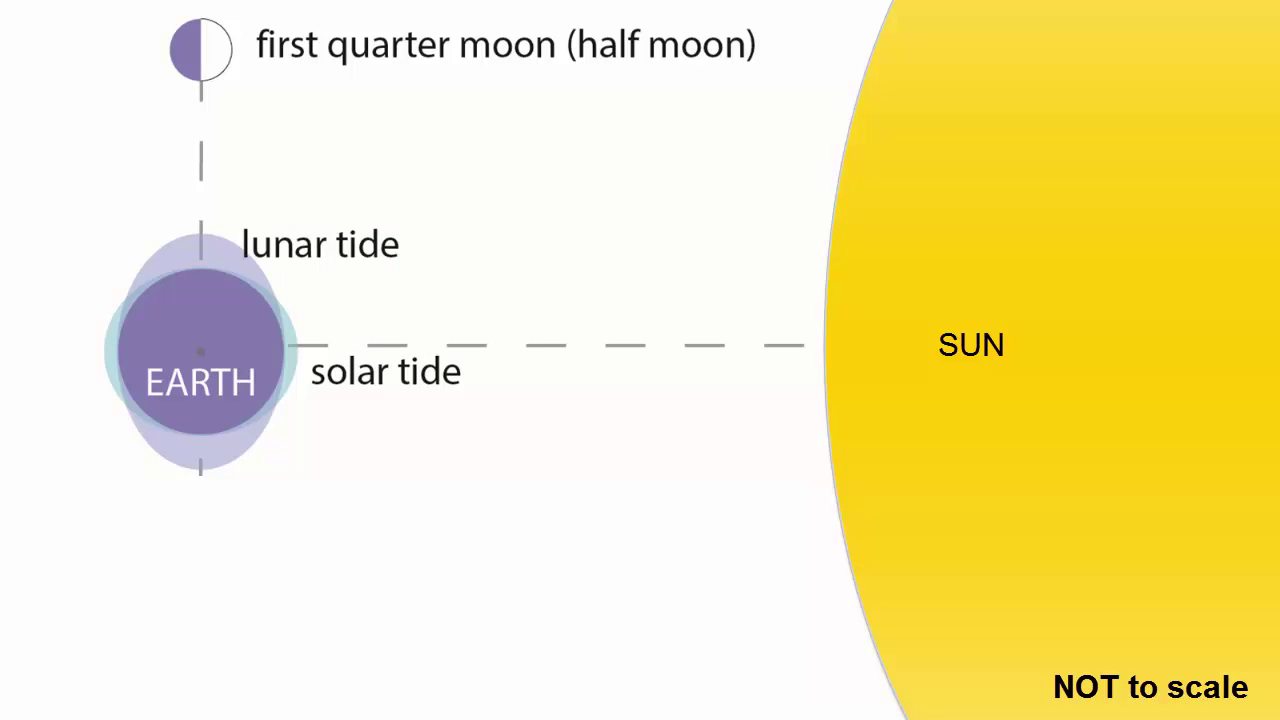
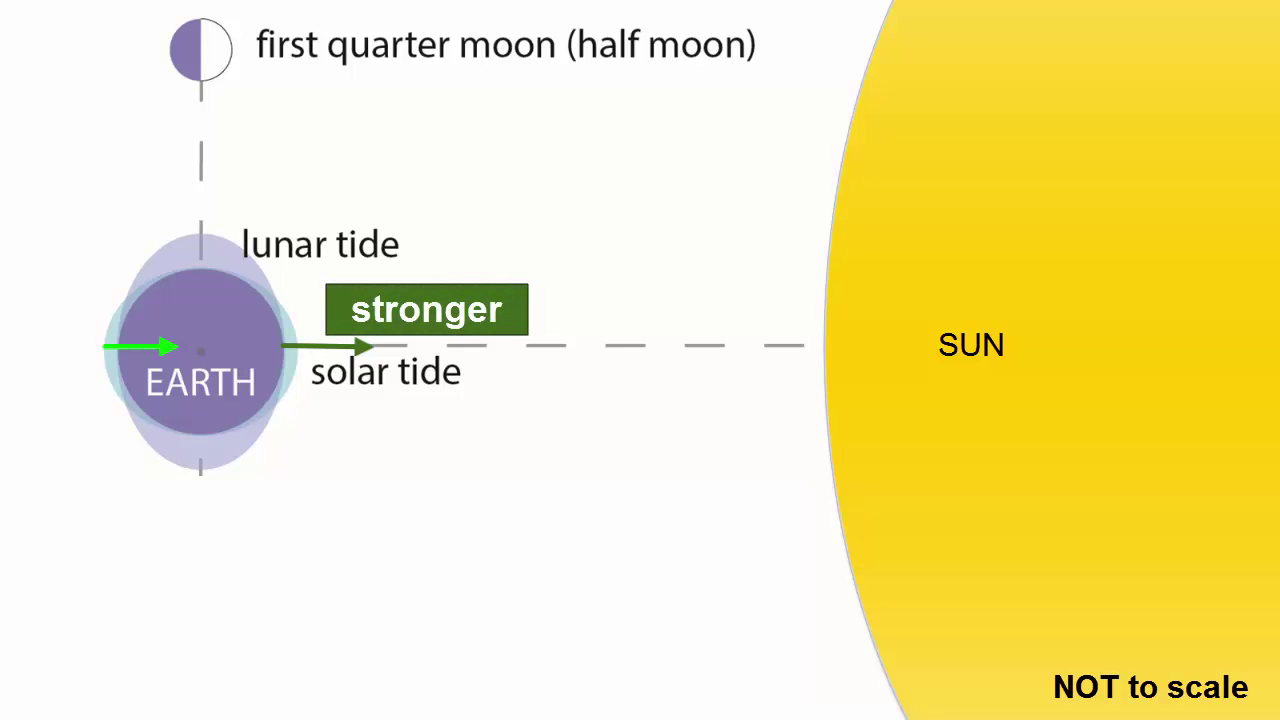
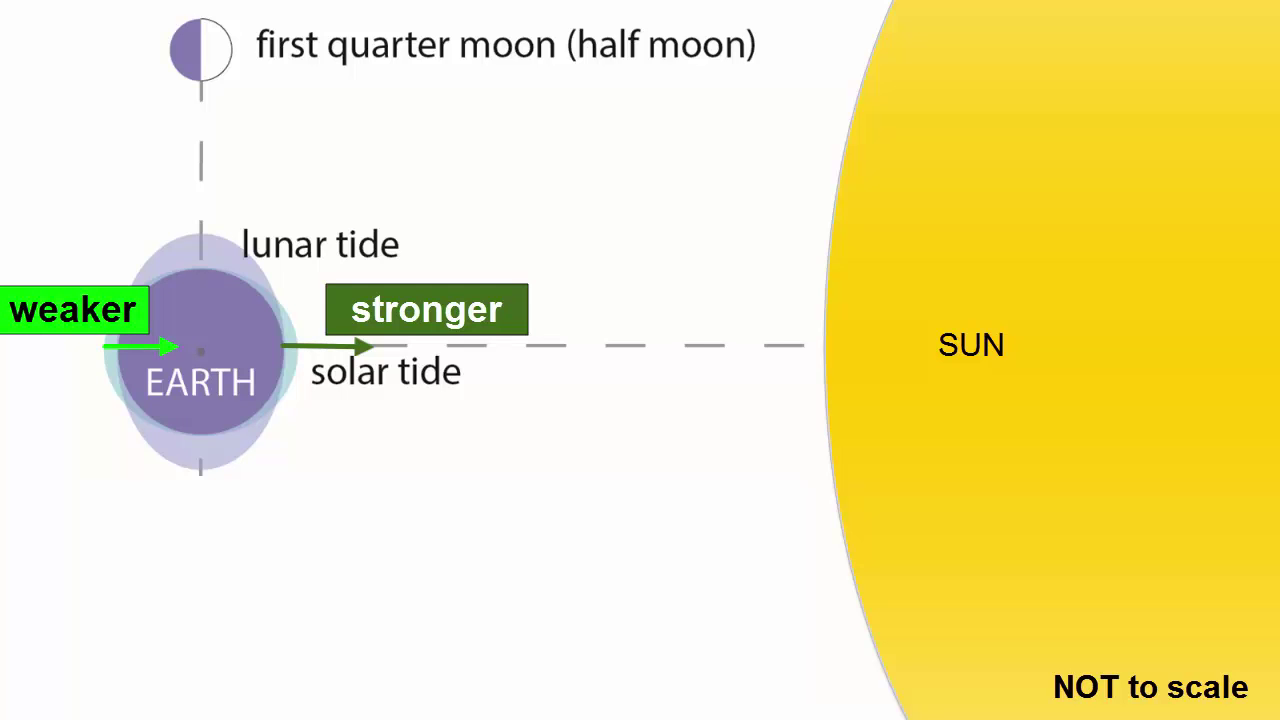
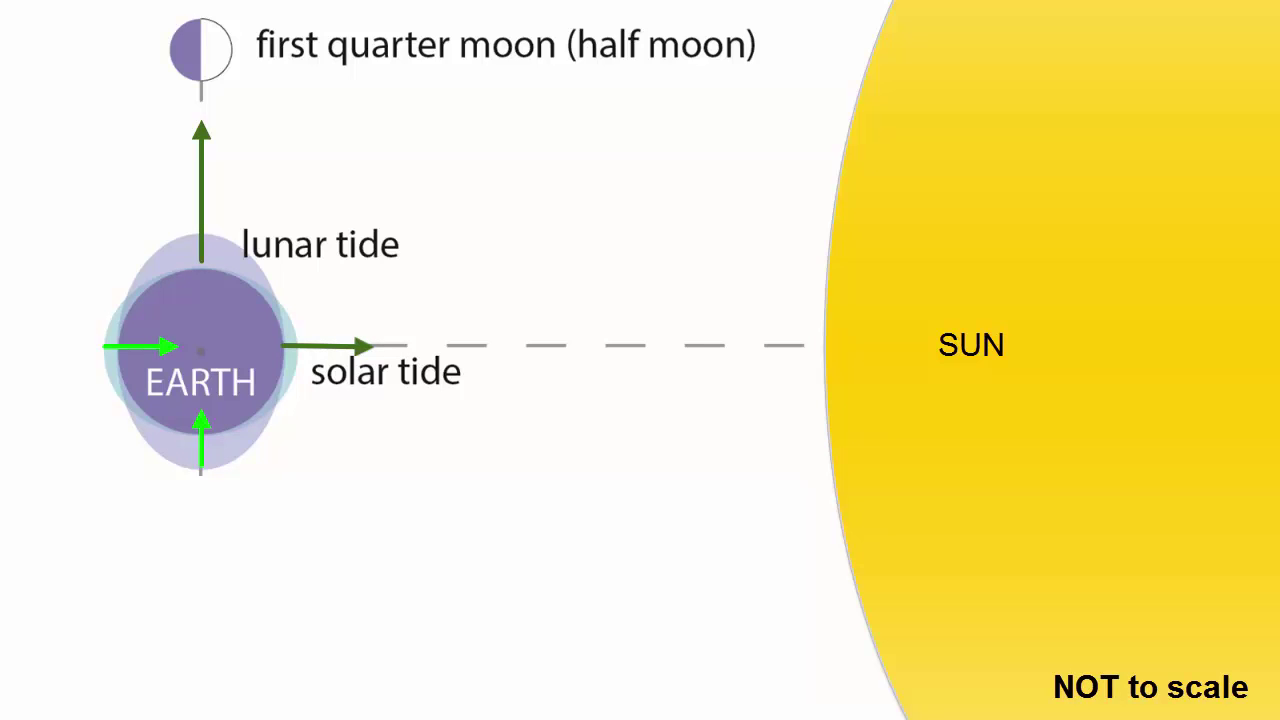
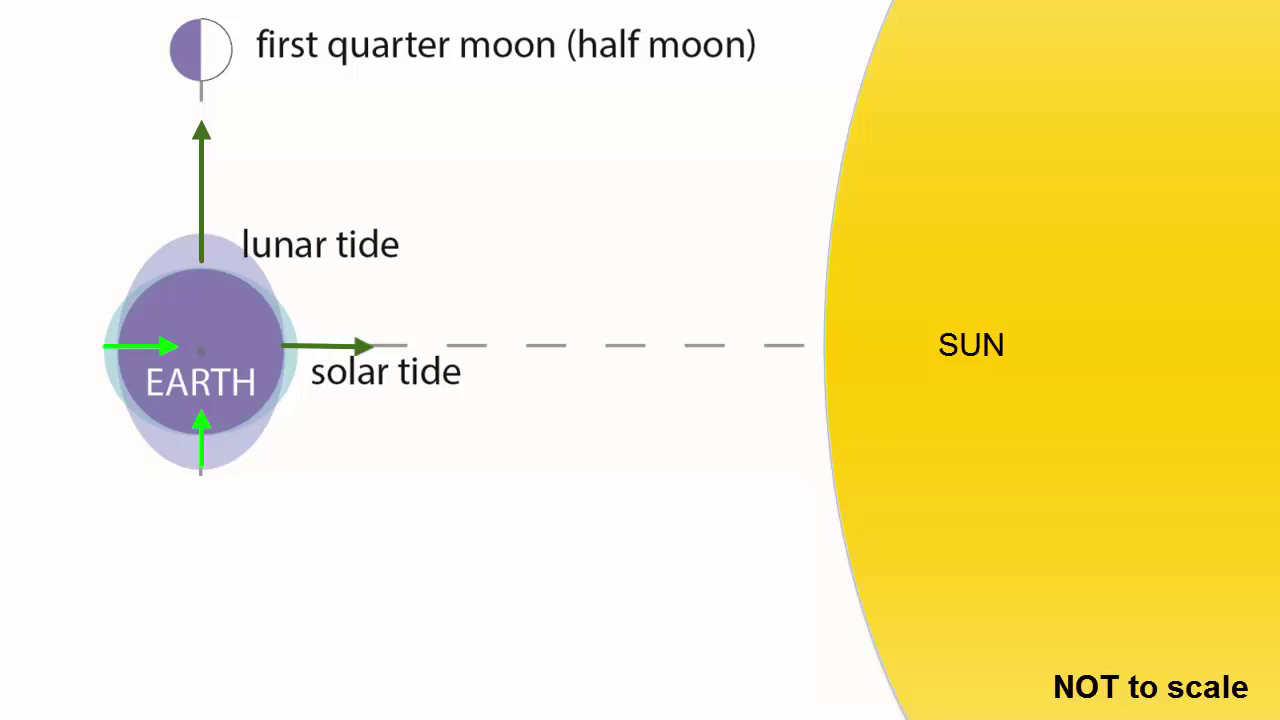
[00:06:44]
Let's return now to the concepts of tidal period and lunar day, as discussed at the start of this tutorial. How does lunar day impact the way we experience tides? This picture shows the Earth's rotation from the point of view of the North Star. Polaris looking down. Notice the stick figure that is starting under one of the bulges, experiencing high tide on the side of the Earth facing the moon. Six hours and 12.5 minutes later, that figure has rotated out of the high tide. Ebb current has removed water from the land, and low tide has arrived. Then flood current starts as the figure heads into another high tide at 12 hours and 25 minutes. 18 hours and 37.5 minutes later, the figure has left the high tide and is experiencing low tide again. 24 hours later, the figure is back where it started relative to the sun, but the moon has been moving during Earth's rotation. The moon has moved 1/29 of its full orbit. The bulges have moved with it, so the figure needs to rotate another 50 minutes to meet back up with the bulge. And that's why we experienced two high tides and two low tides every 24 hours and 50 minutes. Or more precisely, the period of one wave is 12 hours and 25 minutes. Pause now.
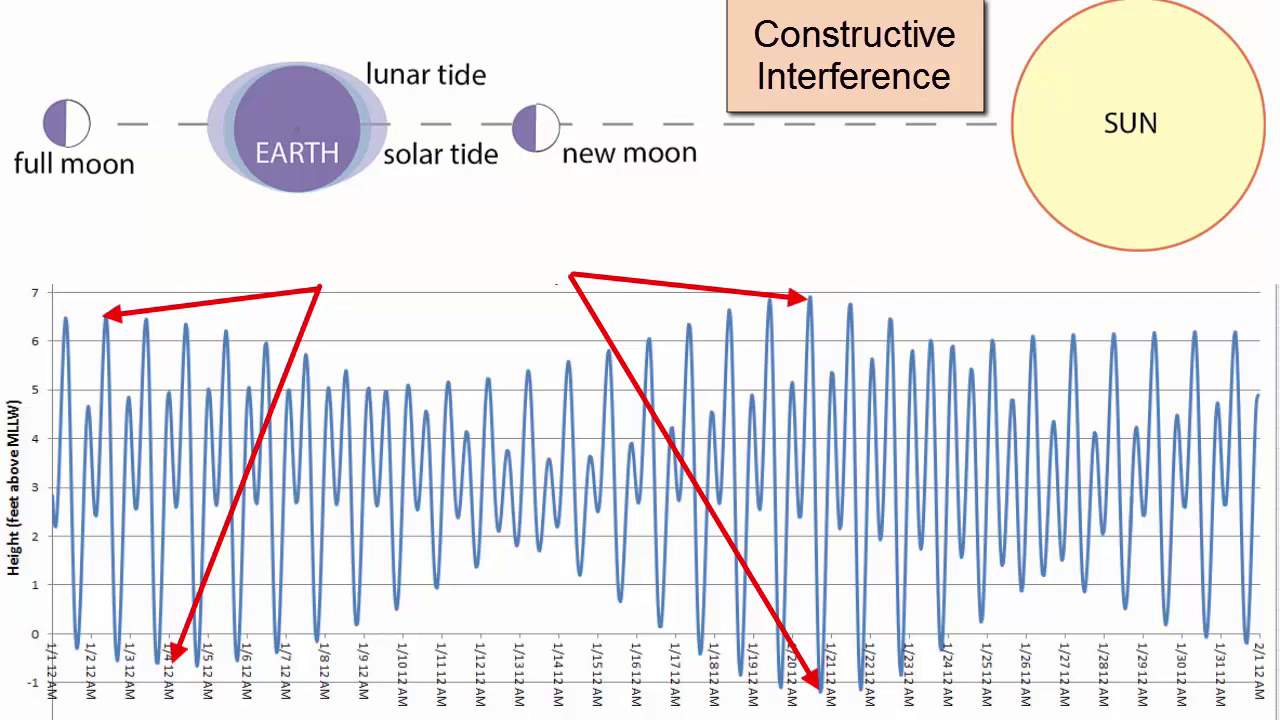
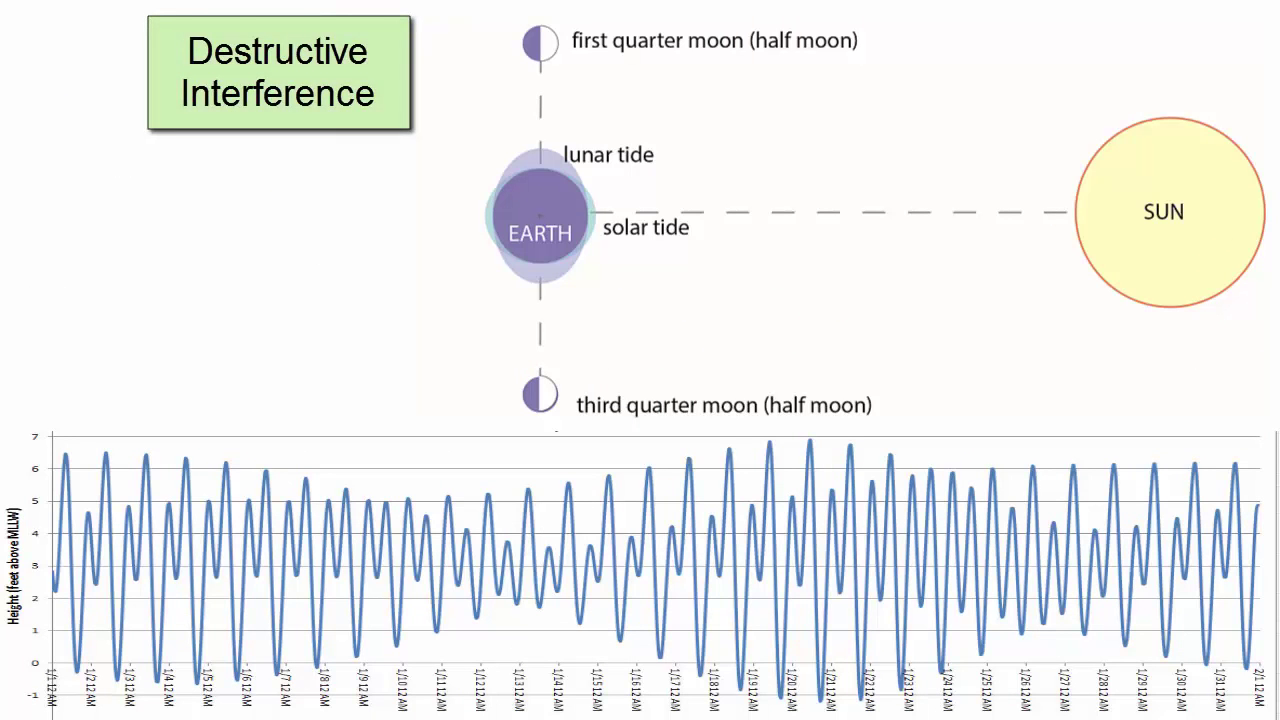
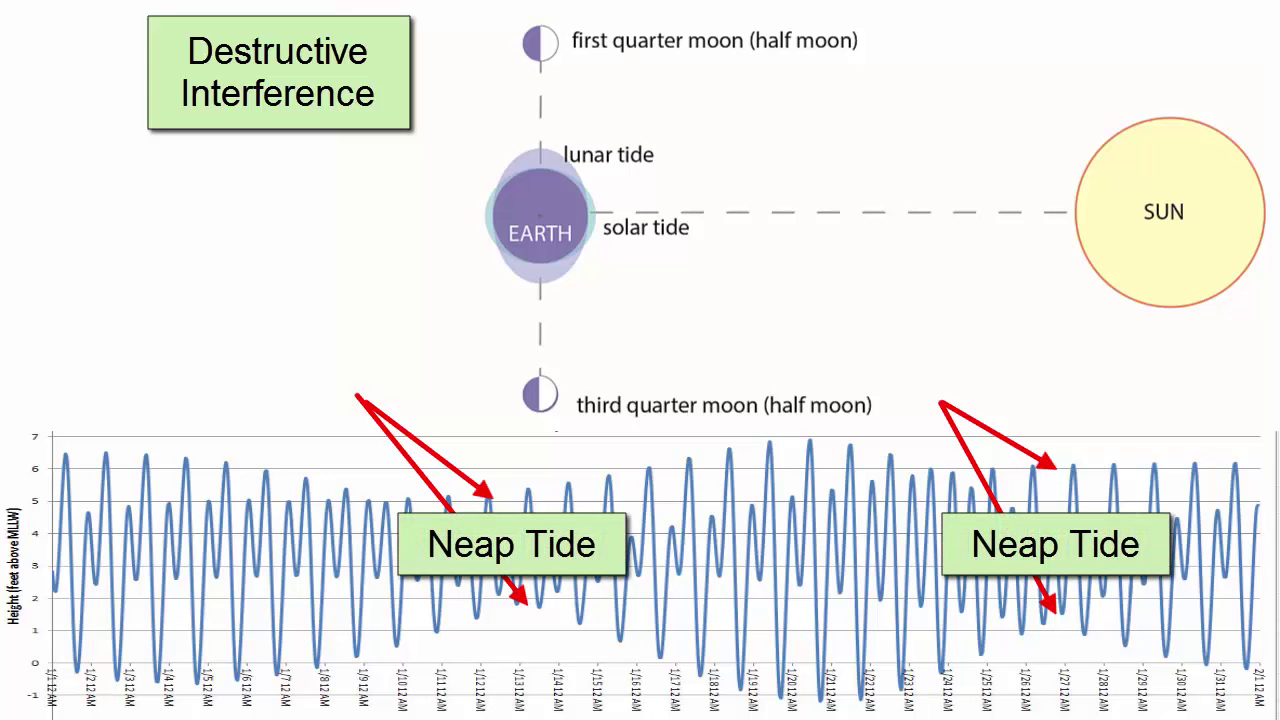
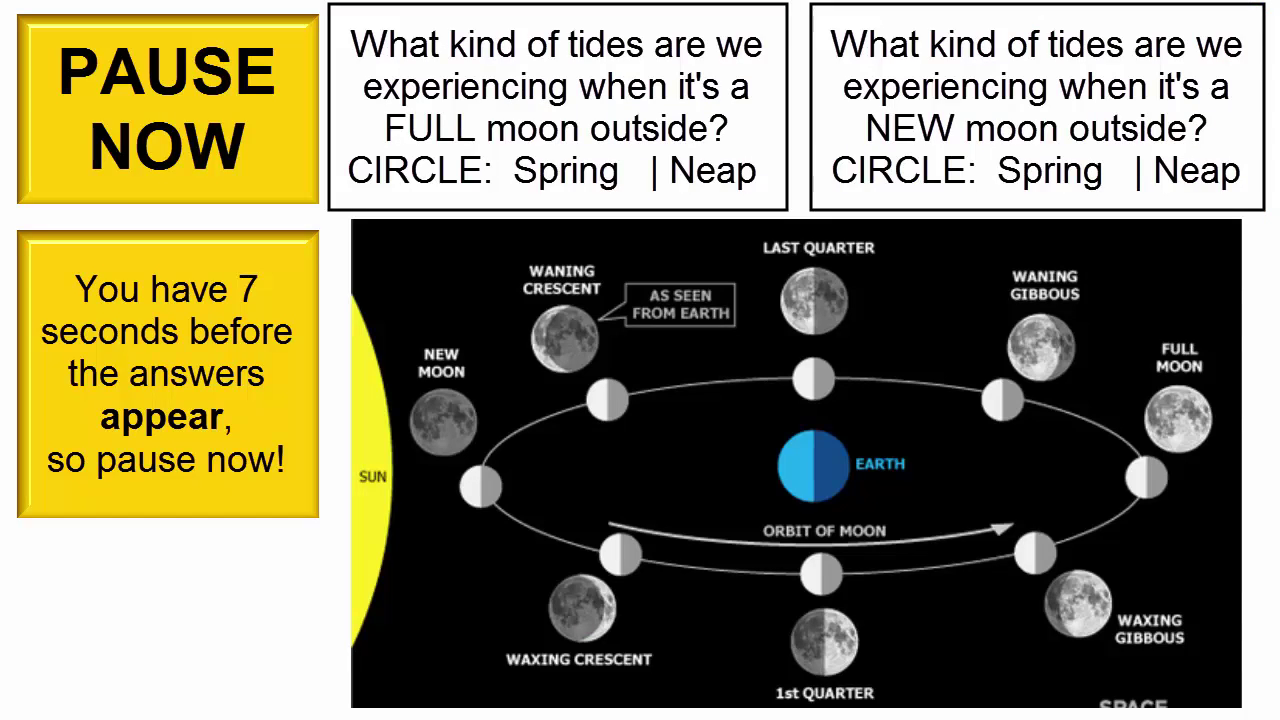
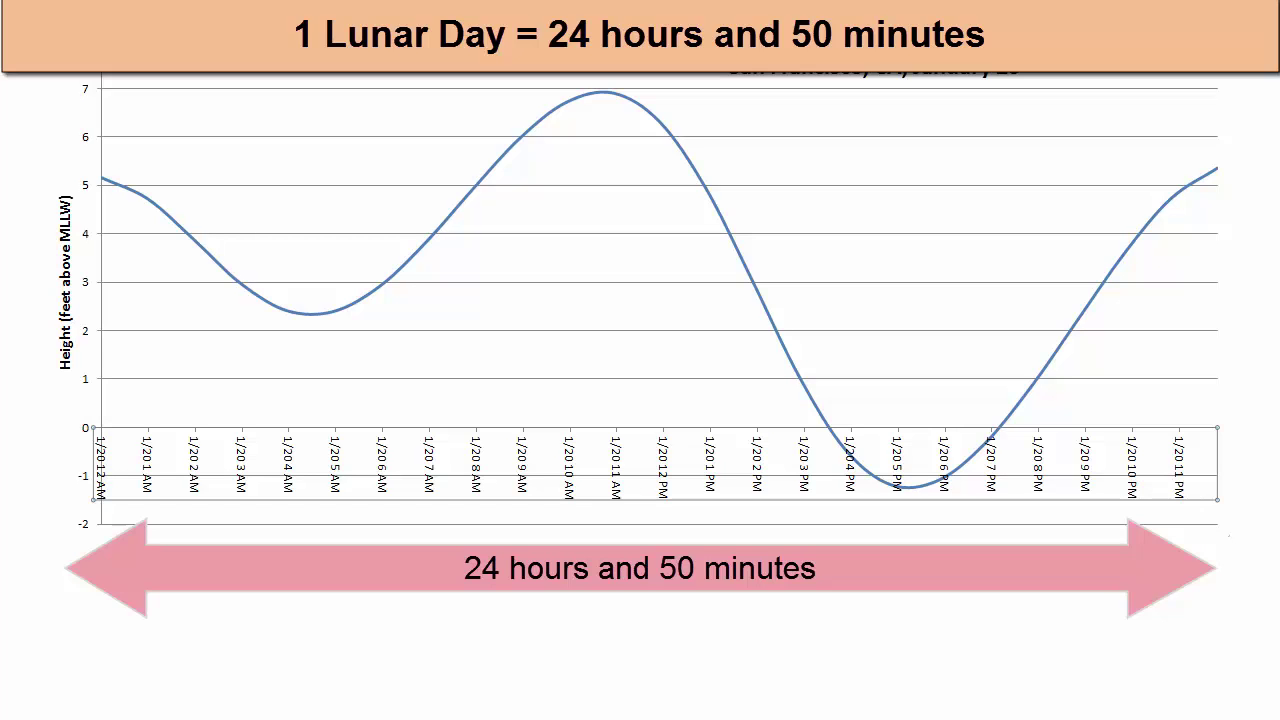
[00:08:18]
How do we use the tidal bulge model to explain the variety of tidal patterns we find across the globe? This picture reminds us that while the Earth is orbiting the sun and rotating through the lunar bulges, it is tilted at a 23.5 degree angle.
So if we were at the equator, we will rotate through two bulges of roughly the same water level and experience semi-diurnal tides. If we are at the mid-latitudes, however, we will move through the two bulges, but different parts of them with different water levels. Thus, we experience semidiurnal mixed tides. At the polls. We miss one bulge entirely, so we rotate through only one bulge per lunar day and experience a diurnal pattern. How does Earth's orbit around the sun affect the tides? Because the sun's orbit is actually an ellipse in January, the Earth is a little closer to the sun than the rest of the year. Remember this cartoon? Imagine it's the sun that's coming closer. What do we get? Greater force differential.
So bigger solar bulges and bigger solar bulges means greater interference with the lunar bulges. More constructive interference when they're in phase and more destructive interference when they're out of phase. The spring tides would have an even greater tidal range, and the neap tides an even smaller one. Now it's all very well to say that the bulges stay in place and the Earth rotates under them. But we know that the oceans are confined to basins, and water doesn't hop over the continents as the planet rotates. How do ocean basins impact tidal behavior? This image shows the Earth with a cut out ocean basin. Since the bulges of water are confined to these basins, they are forced to slosh back and forth as the Earth rotates relative to the moon in the Northern Hemisphere. As this water sloshes westward, it is deflected to its right by the Coriolis effect, which means it sloshes west and north, where it hits and moves along the northern edge of the basin until it hits the western boundary, where it's then forced southward along the edge of that boundary. As it sloshes back eastward, it is again deflected right, which means it sloshes up and along the southern edge of its basin. Combined together, this sloshing around the edges of the basin creates a rotary wave with a 12 hour and 25 minute period, much like water sloshing around the outside of a bucket counterclockwise in the Northern Hemisphere and clockwise in the Southern Hemisphere.
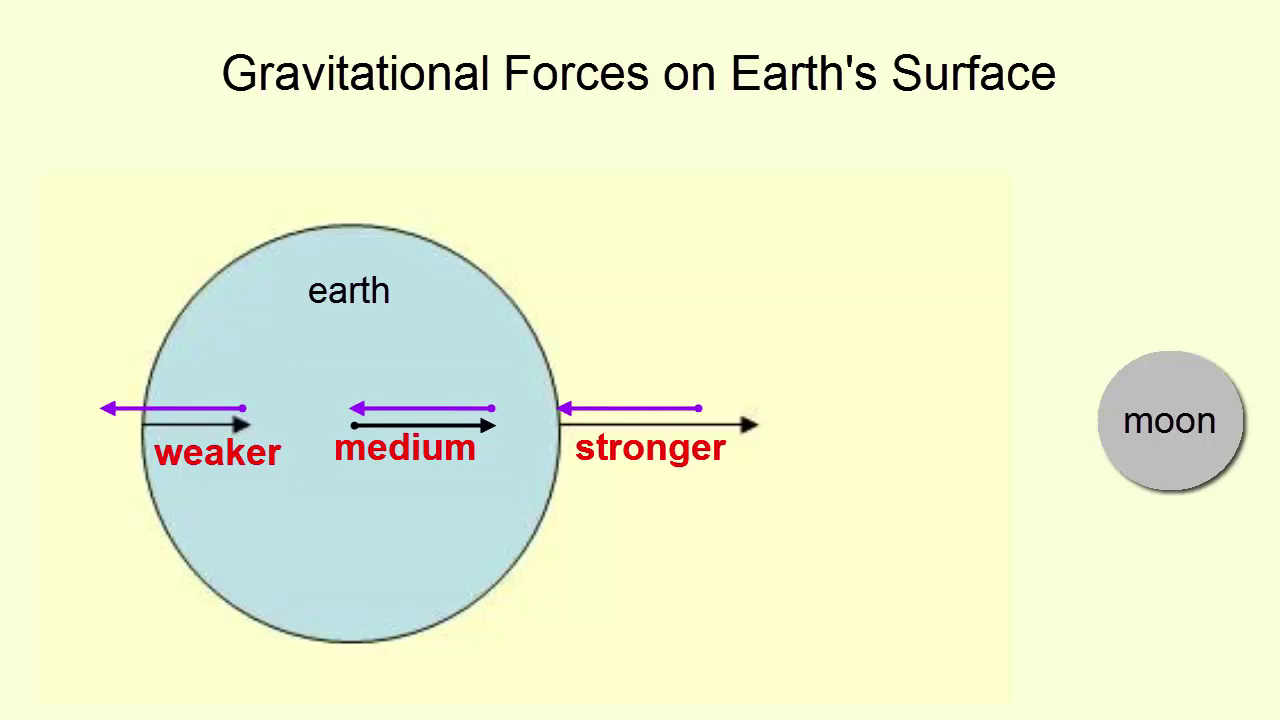
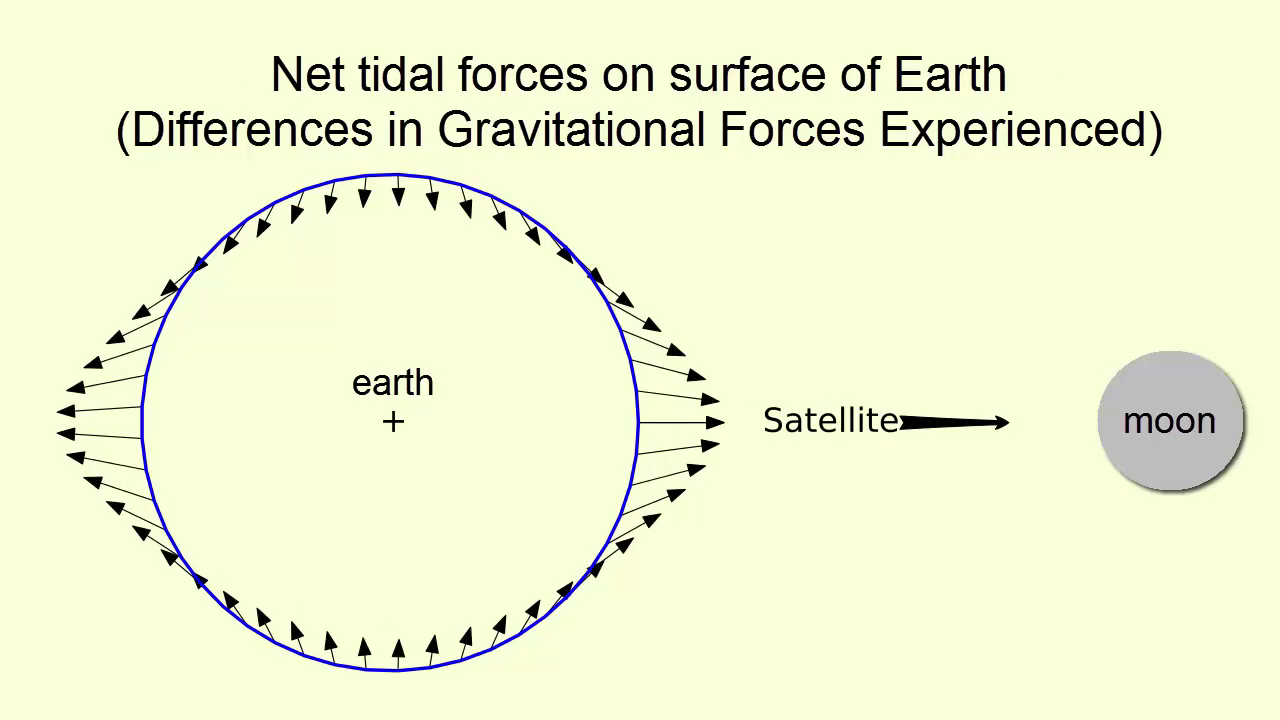
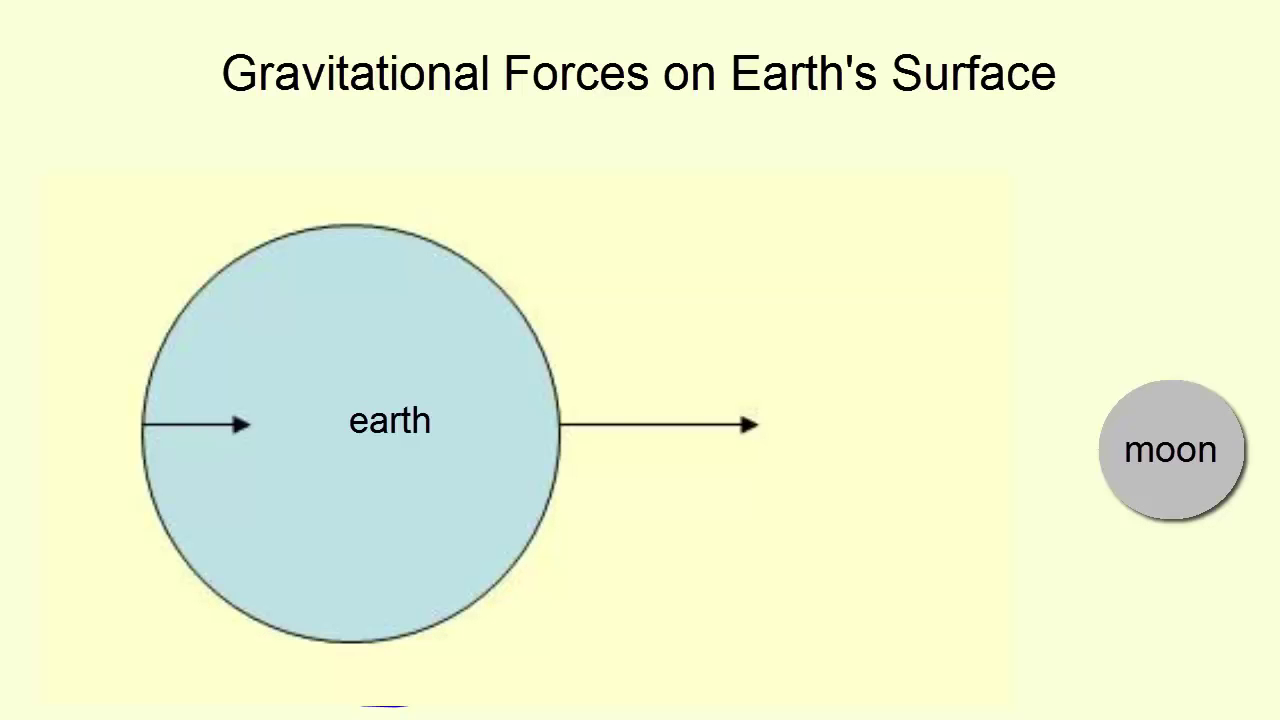
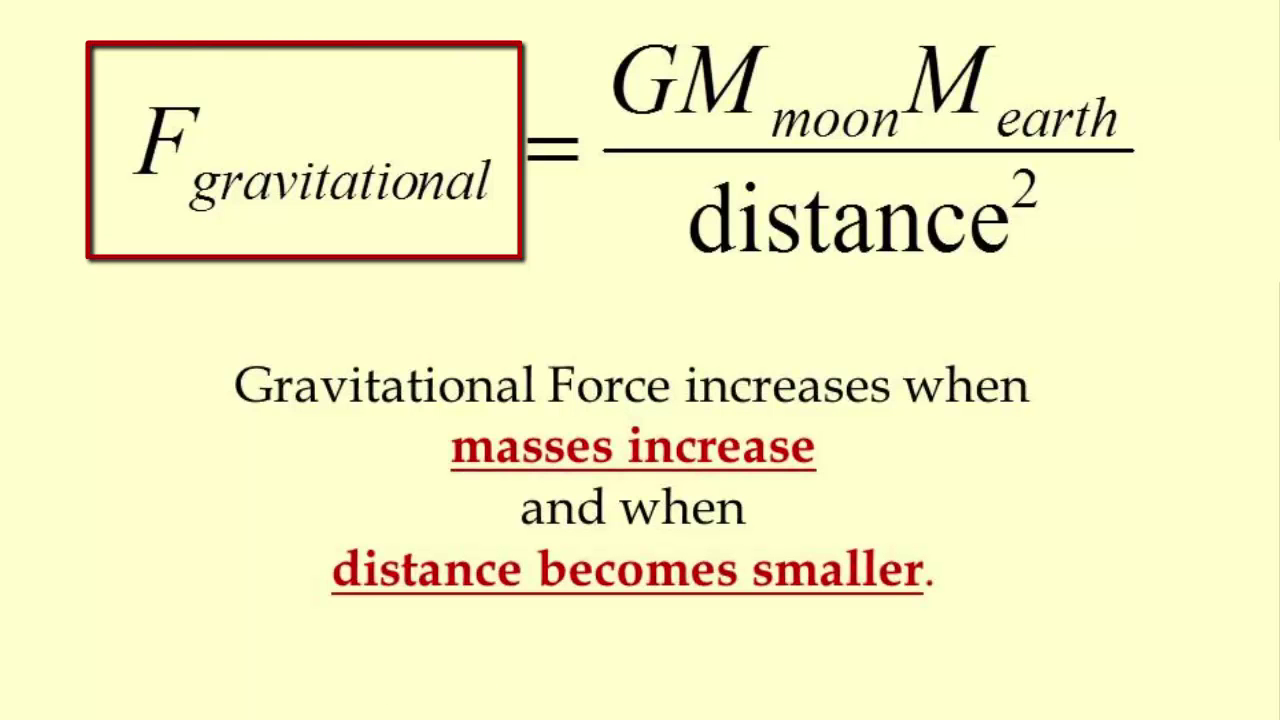
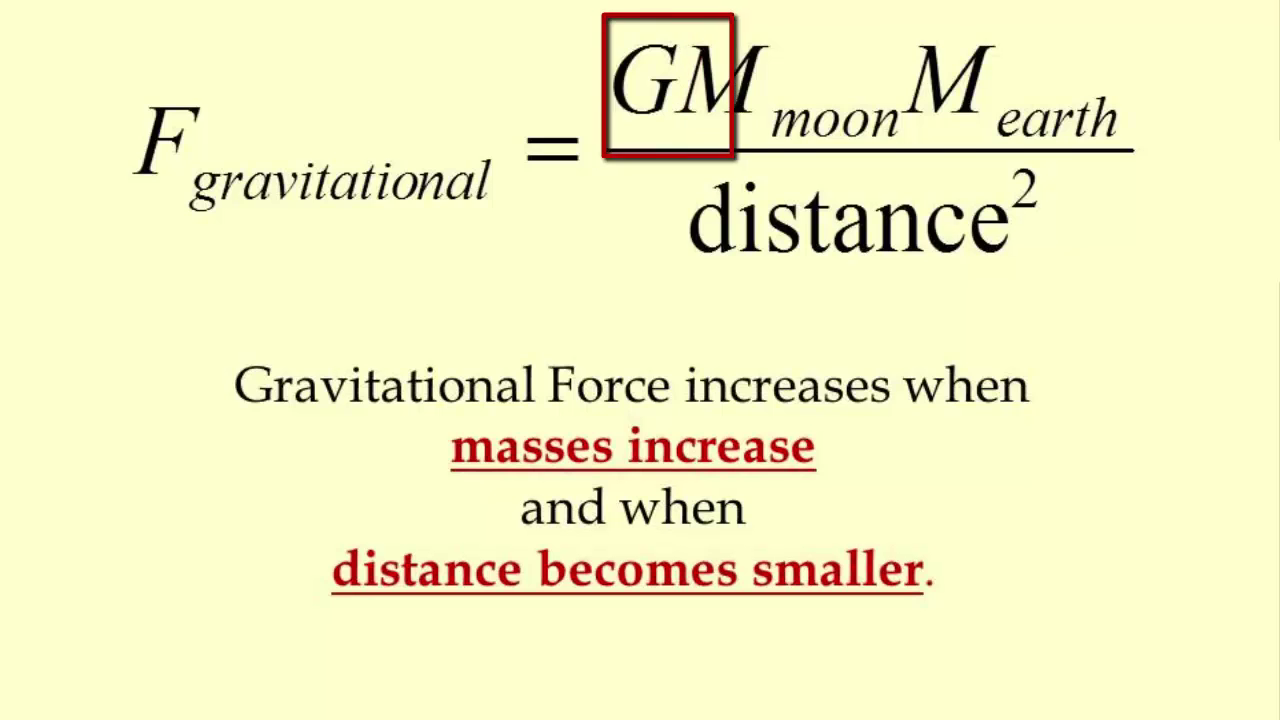
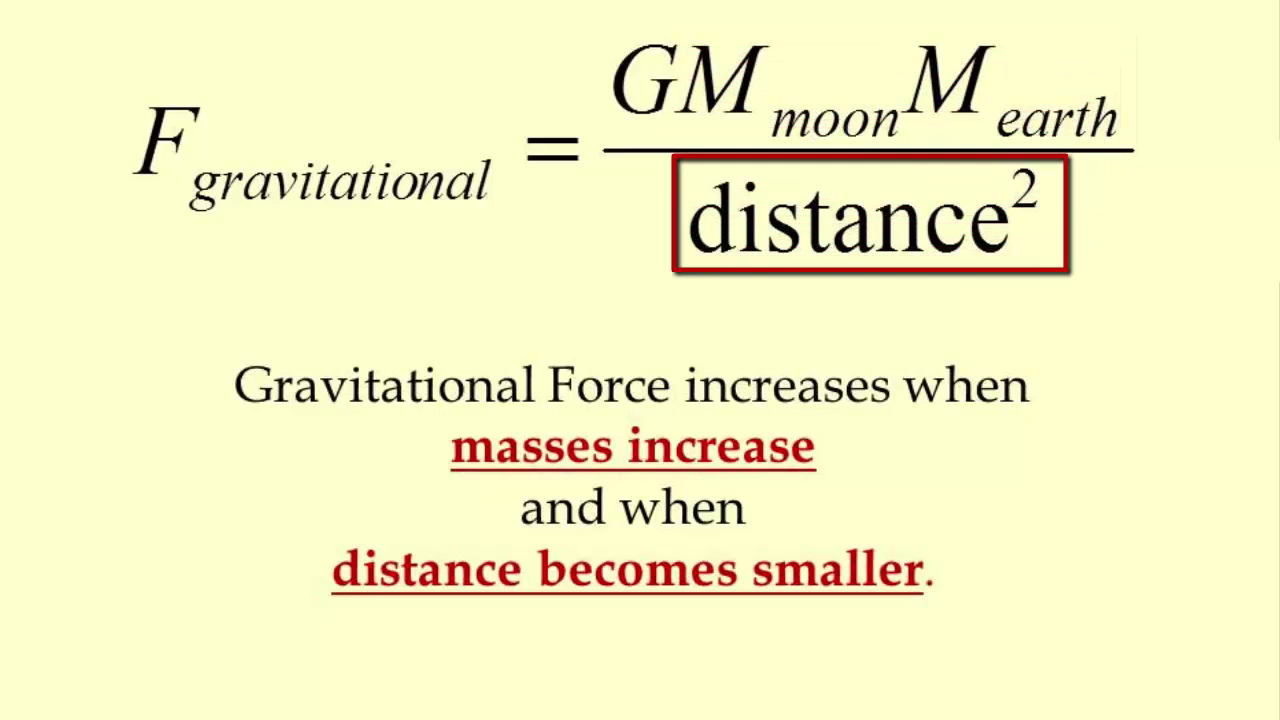
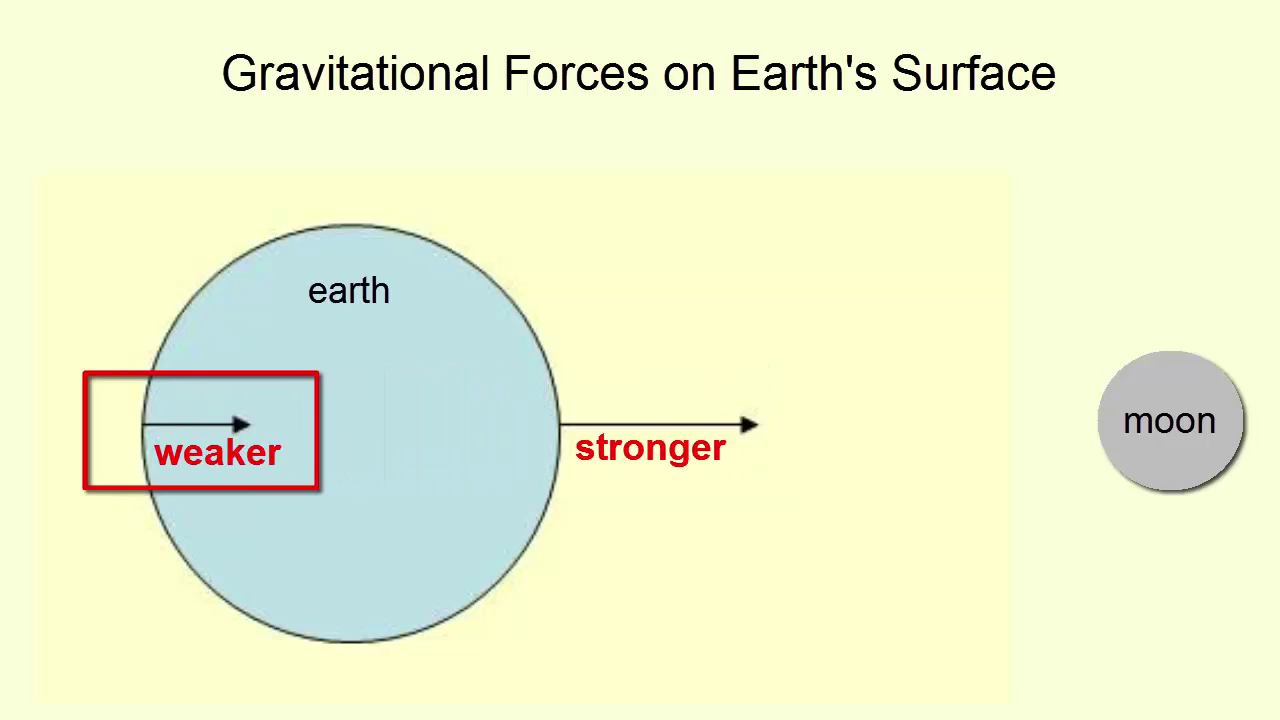
this results in repeating oscillations of a diurnal and semidiurnal tides, where at the same latitude, highest high tides rotate with lowest low tides to form a concurrently rotating system resulting in extremes of at higher latitude, the big high tide would be no bigger than the low tide, so... one tide per day. (all because of the tilt in the plane of the earth's orbit)
twice a month stronger tides and twice a month weaker tides
This just shows when the new moon intercedes with the sun you get a strong tide (Spring tide)
a week later, the solar and lunar (growing half moon) tides are canceling each other out,
but since the lunar tide is twice as strong as the solar tide there wont be zero tide, but as weaker tide. a week later a full moon reinforcing again for a stronger tide
adding another oscitation that has to be accounted for is the lunar orbital period
this motion also adds a dimensional wobble to earths orbit
The Phases of the moon also change on a period of 29.5 days . which dictates a change in maximum tidal amplitude. the time between a new moon and a full moon, the time between these maxima of tidal amplitude of 15 days
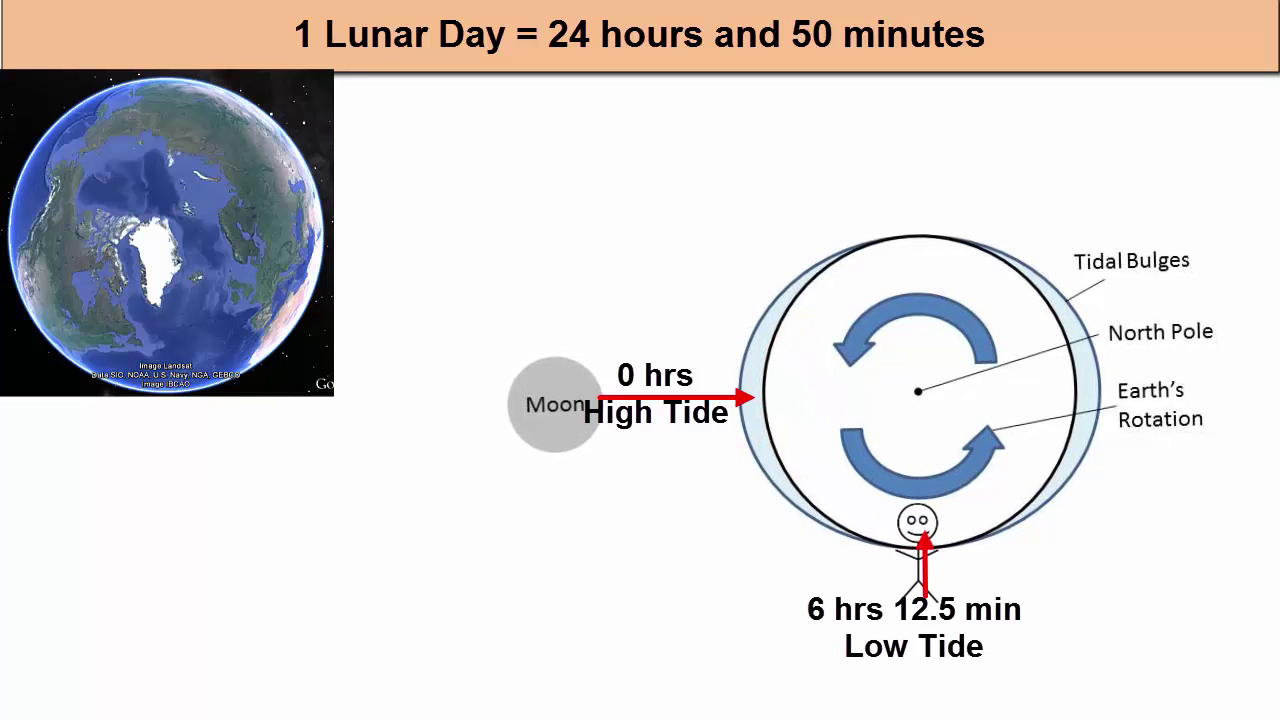
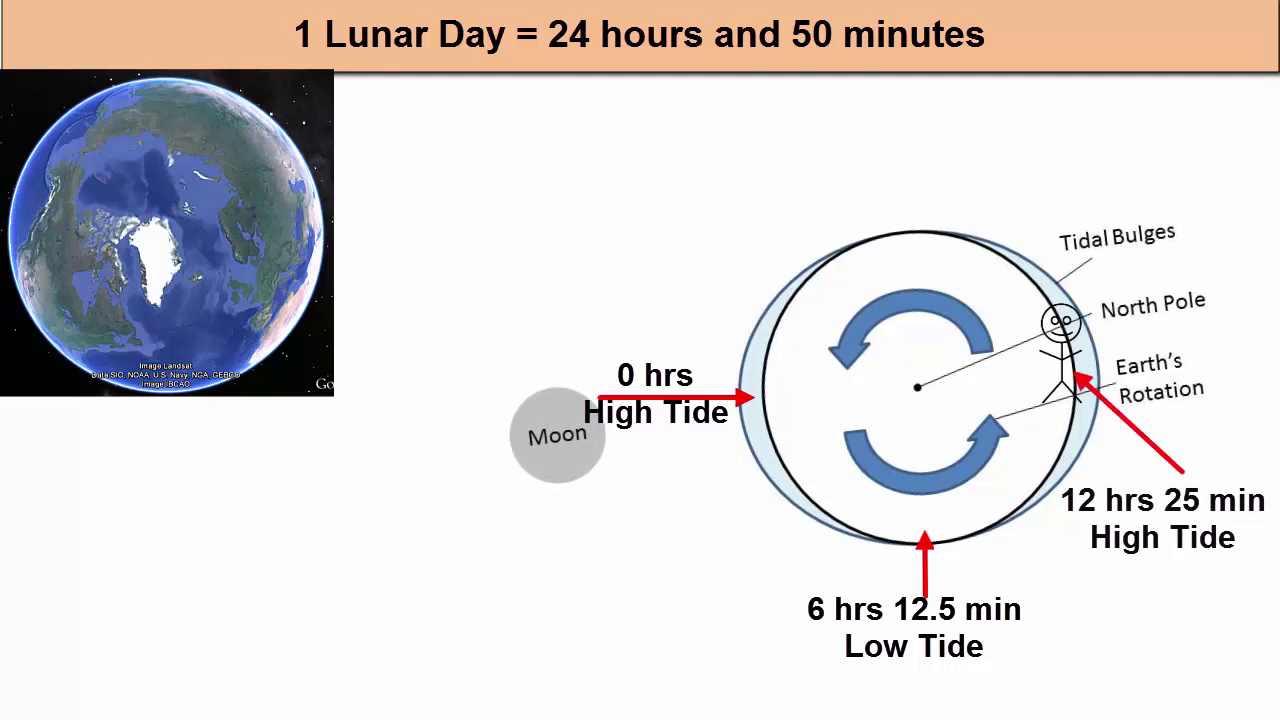
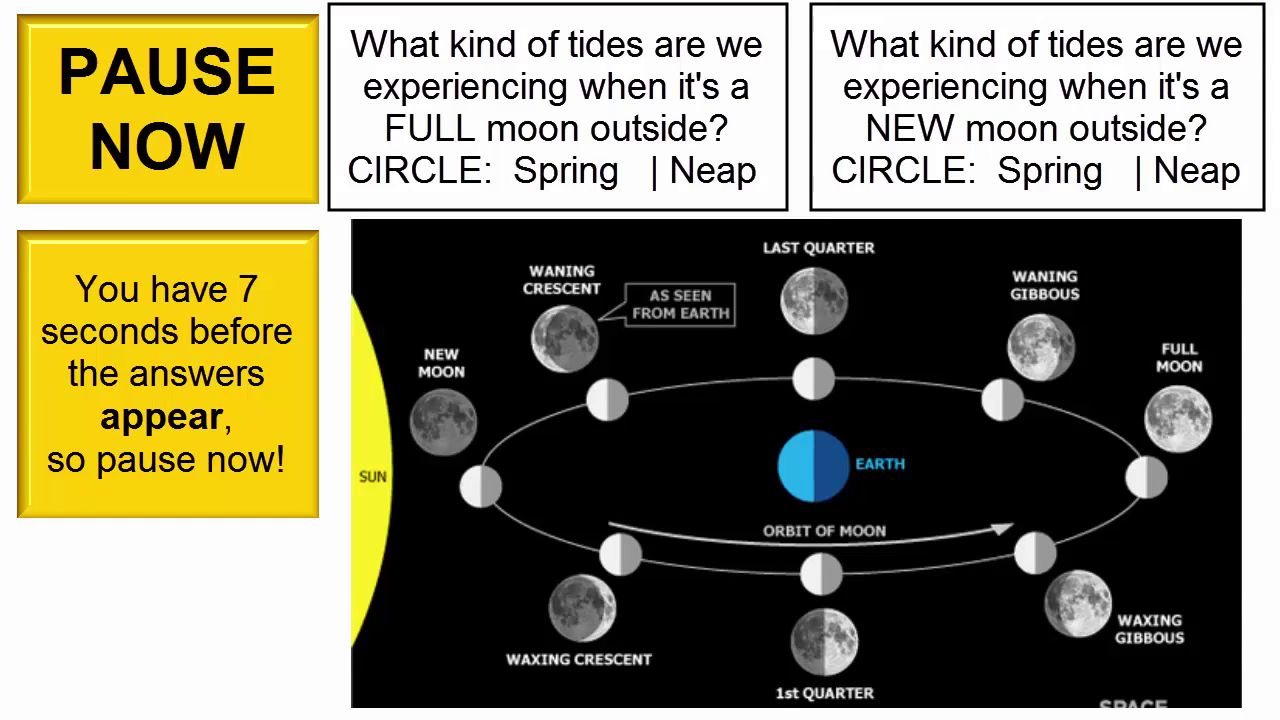
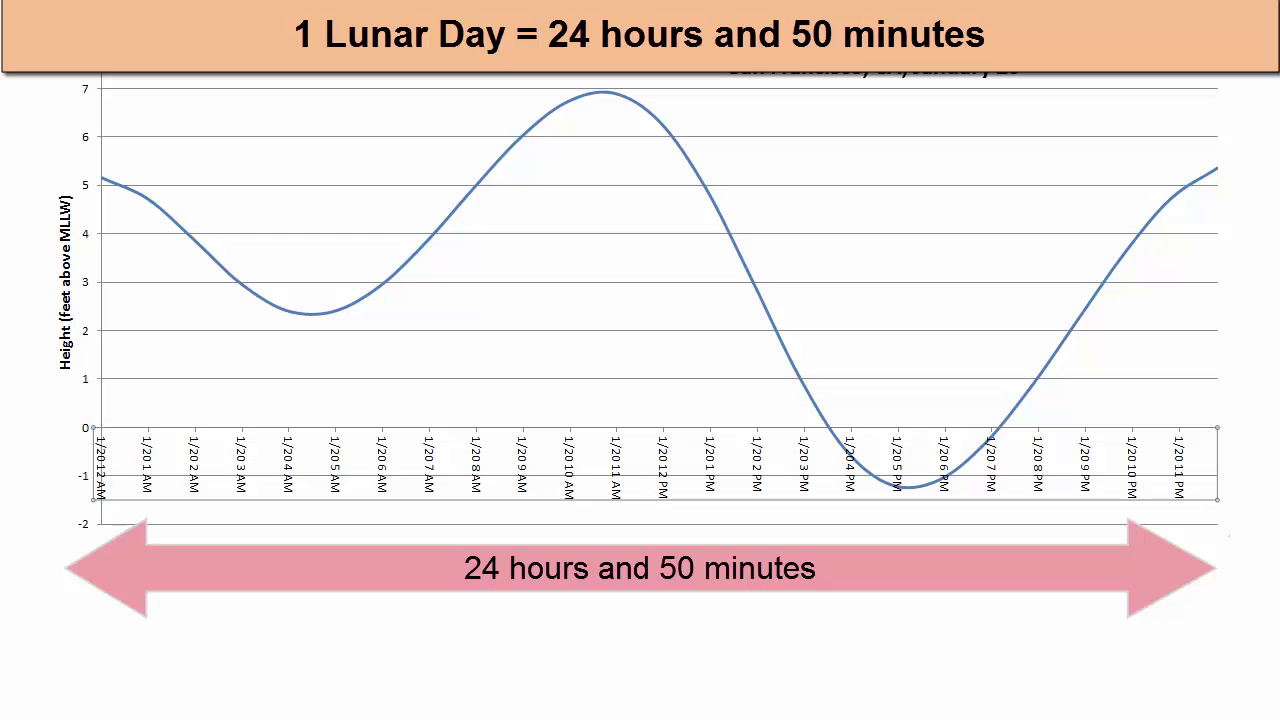
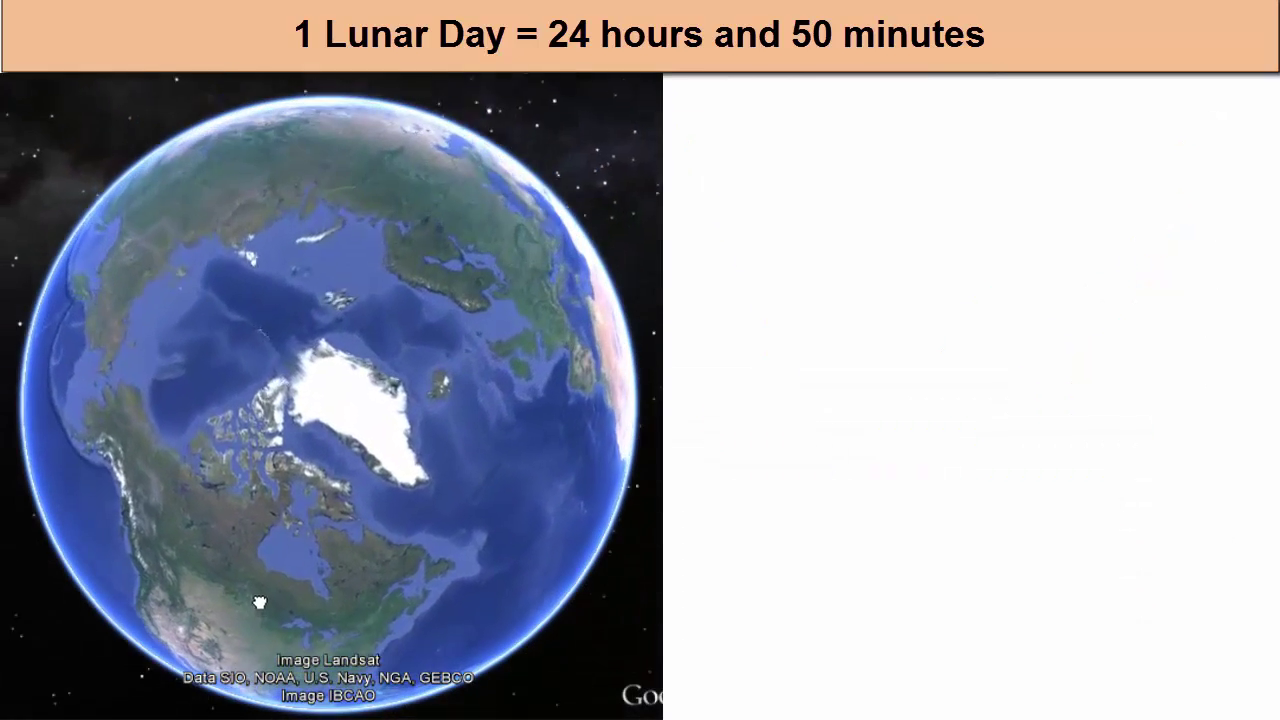
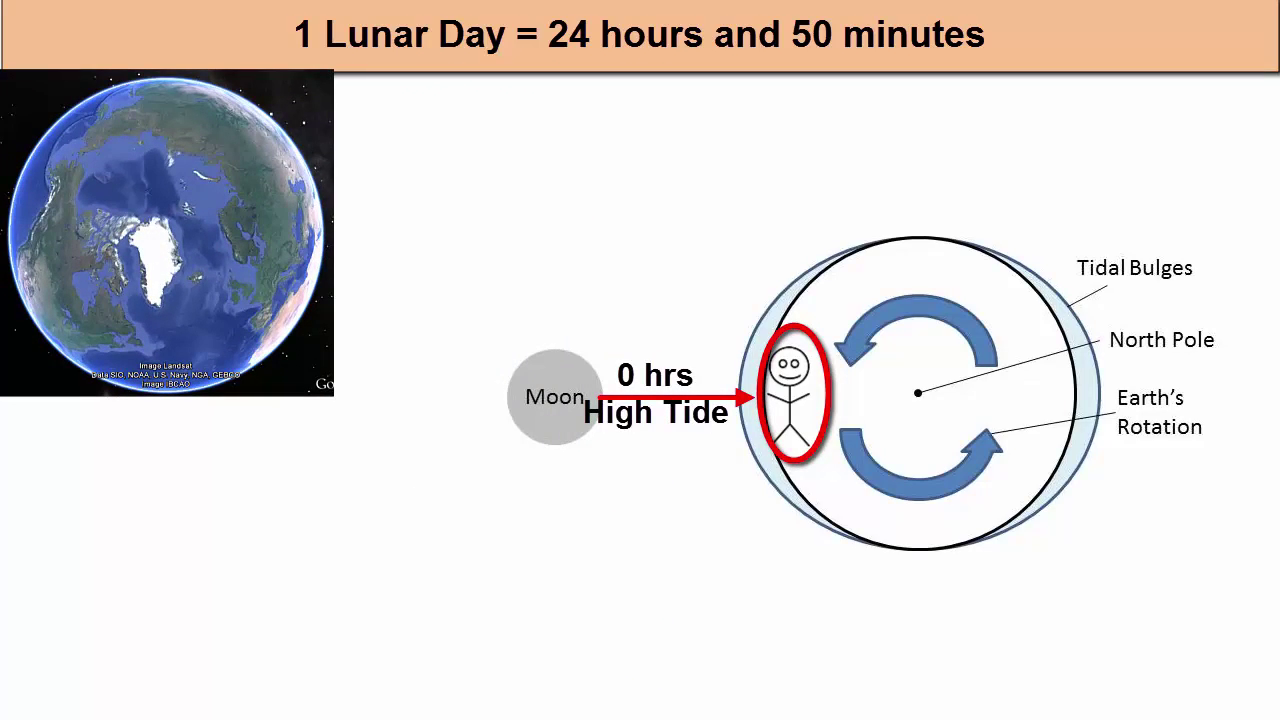
We call each ocean basin around which tides slosh and amphidromic system. In the center of each bucket of sloshing water, there's a point where the water level doesn't change. We call that the amphidromic point. Around the amphidromic point, the tides rotate once every 12 hours and 25 minutes. This cross section through the Amphidromic system points out what the sloshing looks like from the side at two moments in time, one where one side has water sloshing up high tide and the other water sloshing away low tide, and then the opposite. Notice that the tidal range increases as we move away from the amphidromic point. So where in this image would you expect to find the largest tidal range? As far away from the amphidromic point as you can get. In this bird's eye map view, we can further describe the Amphidromic system with lines and circles. These lines, that look like spokes of a wheel, represent the location of the high tide every hour as it moves around the bucket. We call these lines cotidal lines because every location along the line experiences high tide or low tide at the same time. The concentric circles are called co range lines because everywhere along each circle locations are equidistant from the center of the bucket, the amphidromic point, and therefore they experienced the same difference between high tide and low tide, or the same tidal range. Let's look more closely at actual data of amphidromic systems from across the world's oceans. Notice this system in the northern hemisphere off the coast of California. First, we see that the tides rotary motion isn't always moving at the same speed. How do we know? Cotidal lines are sometimes close together, other times much further apart? Why the difference in speed? As we discussed in the waves video tutorials, waves speed up in deeper water and slow down in shallower water. So the speed of the tidal waves here are telling us something about the depth of the water. We also see that due to the counterclockwise movement of tides in this system, the tides move up the coast of California northward. So if you have a friend in LA and she says the high tide has just arrived at her beach, then you can expect it to arrive at Ocean Beach off San Francisco about two hours later. Areas with the largest tidal range can be found further away from the amphidromic points, and those with the least tidal range are closest to them. There are many amphidromic systems across the world's oceans, produced by the varying shapes of the ocean basins and the configuration of continents and islands. Let's piece together again how you would draw an idealized amphidromic system in a square ocean basin in the Northern hemisphere. First, you'd start by creating the spokes of your wheel, the cotidal lines all coming out from the Amphidromic point center. Be sure to include enough to get a divisor of 12. Then we can pick a starting point for our wave and demonstrate how it moves counterclockwise. In this case, jumping two hours for each spoke until we get back where we started 12 hours and 25 minutes later. Next we add the Co range lines as concentric circles, and we label them with increasing tidal ranges as we move outward from the amphidromic point.
[00:14:23]
Pause now.
[00:14:35]
For more information and more detail, continue on to the next video in this series.
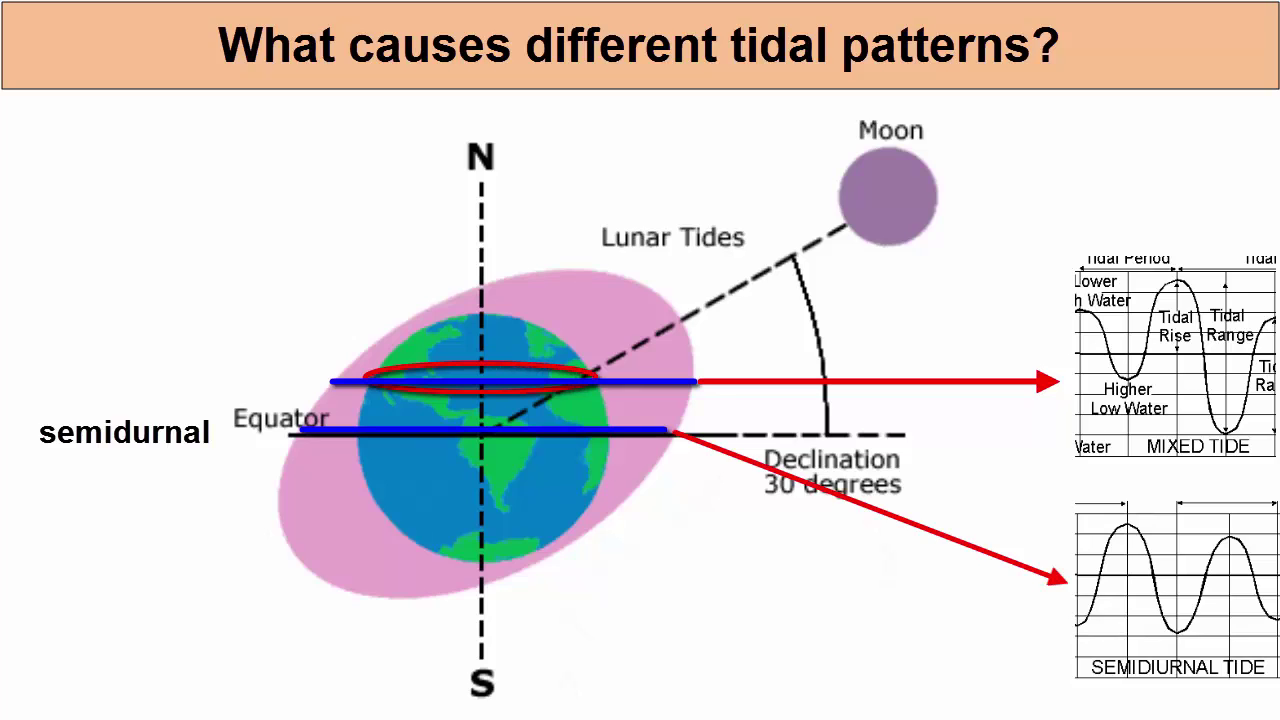
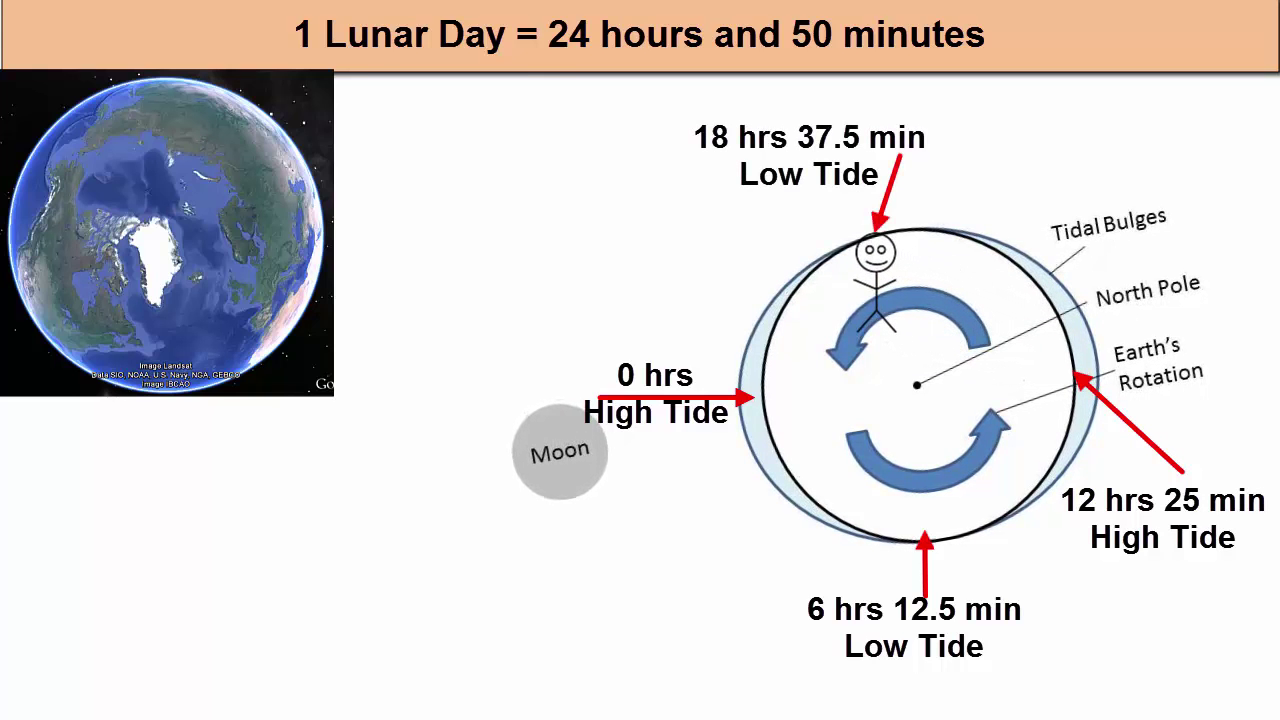
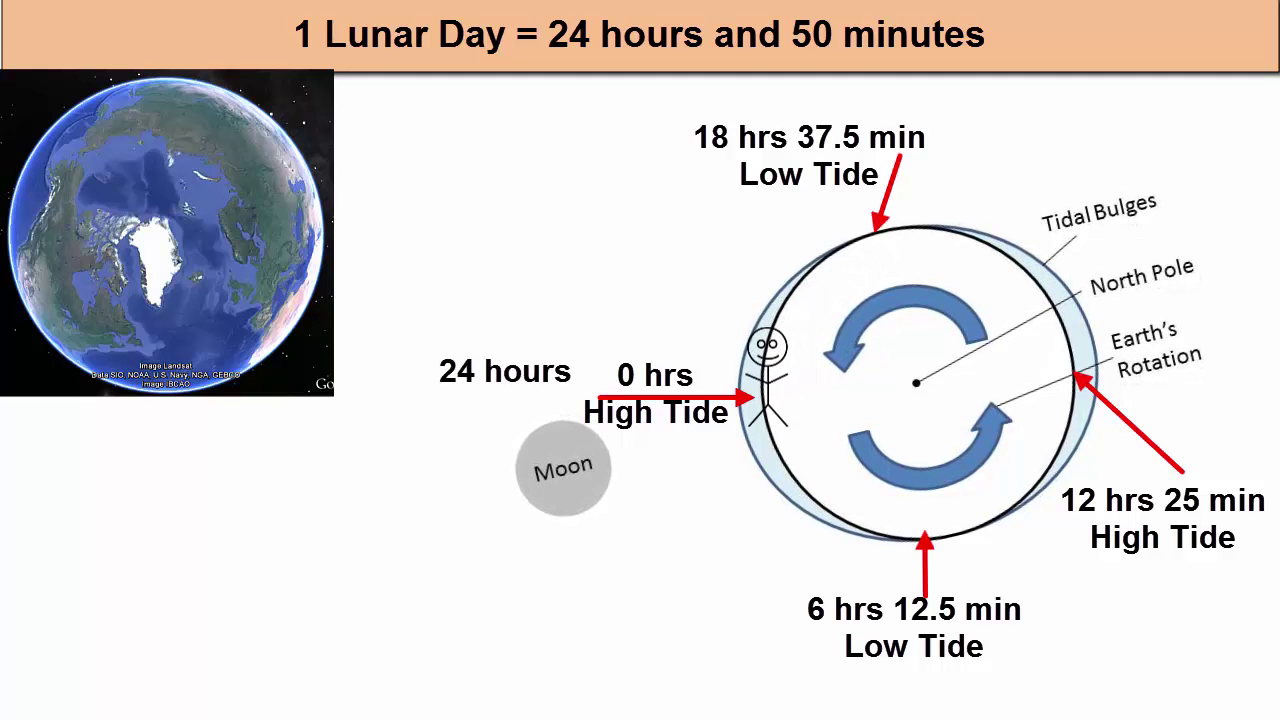
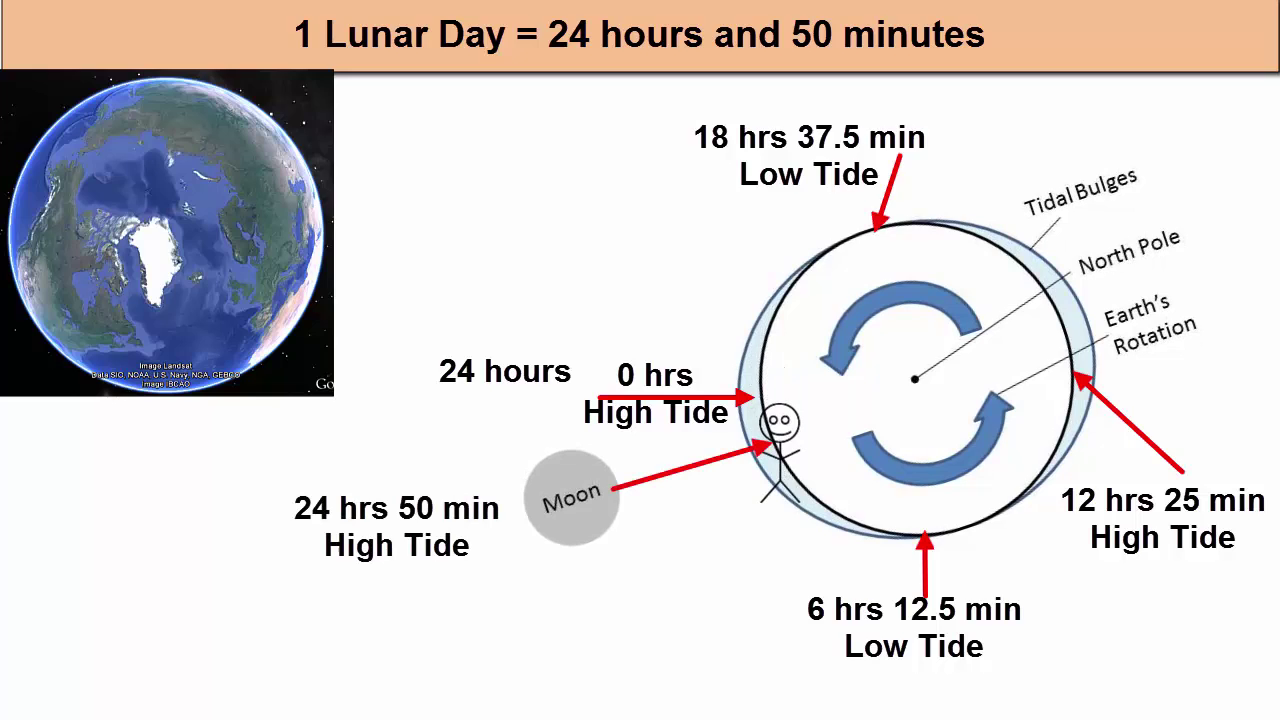
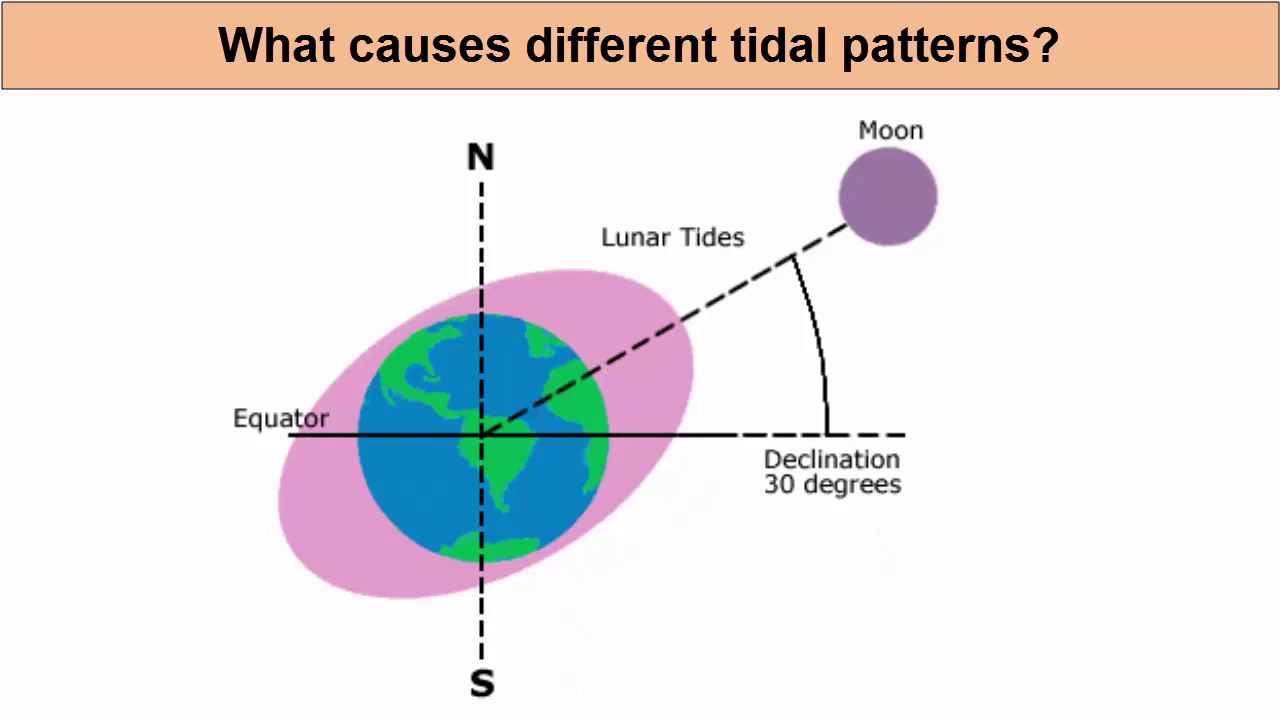
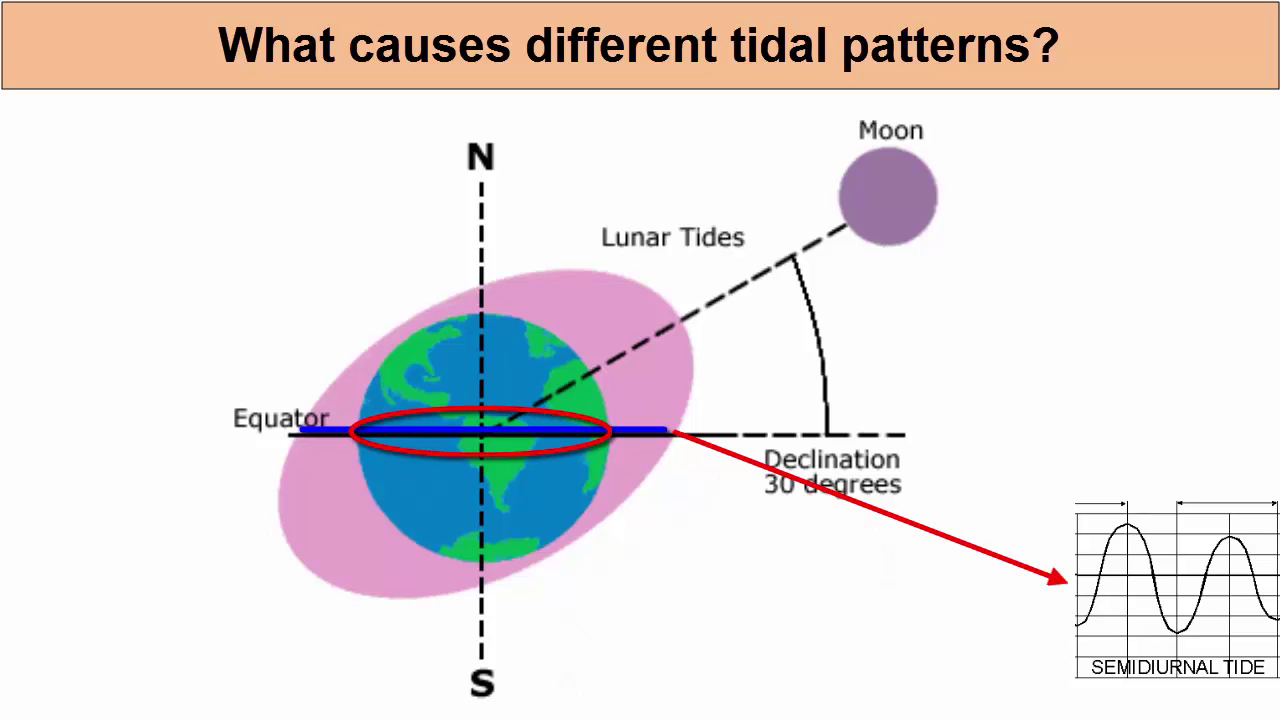
Intro for above:
**[Segment 1: Understanding Tidal Periods: 0:21-1:10]** "Did you know that most tidal waves have a period of one wave every 12 hours and 25 minutes? This coincides with Earth's rotation relative to the moon. After rotating once relative to the sun in 24 hours, Earth needs an additional 50 minutes to align with the moon. This duration is what we call a lunar day. It's a beautiful cosmic synchrony!"
**[Segment 2: Moon's Role in Tides: 1:11-2:00]** "The moon, our closest celestial neighbor, plays a starring role in creating tides. An illustrated image, not to scale, shows Earth being pulled by the moon's gravity. The gravitational force is stronger on the side of Earth closest to the moon, creating a tidal bulge. This force decreases with distance, leading to another bulge on the opposite side."
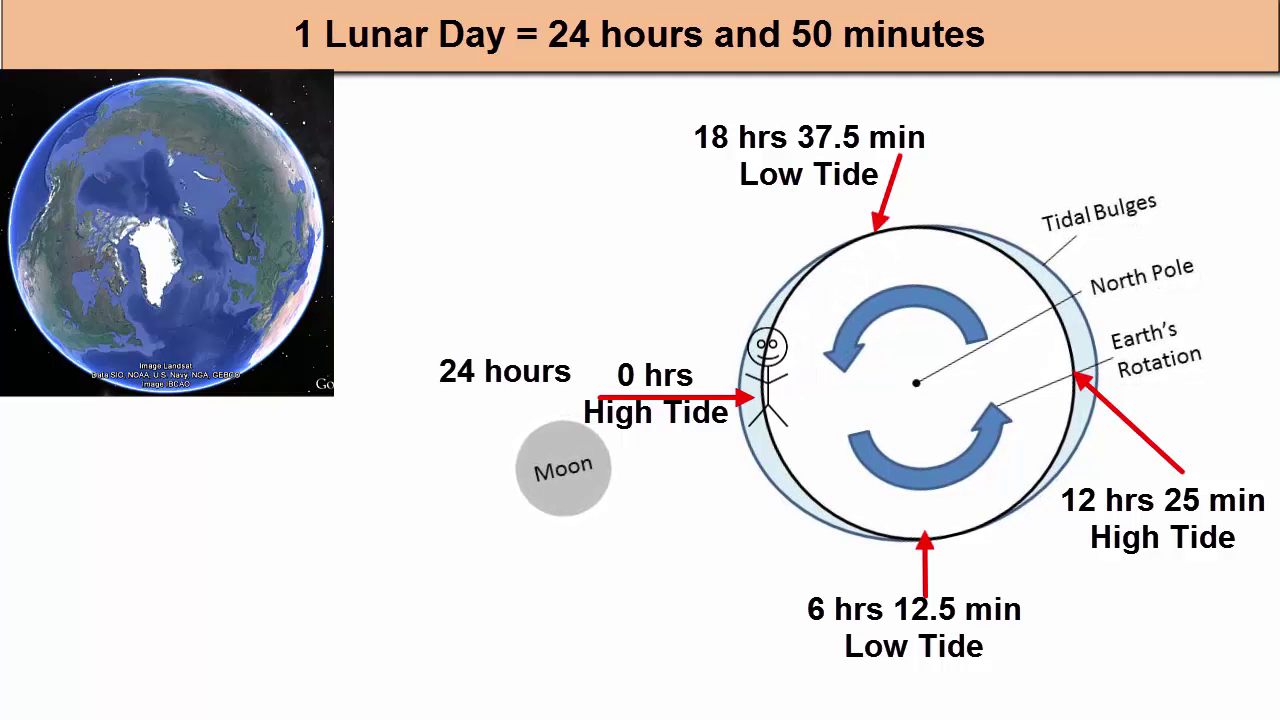
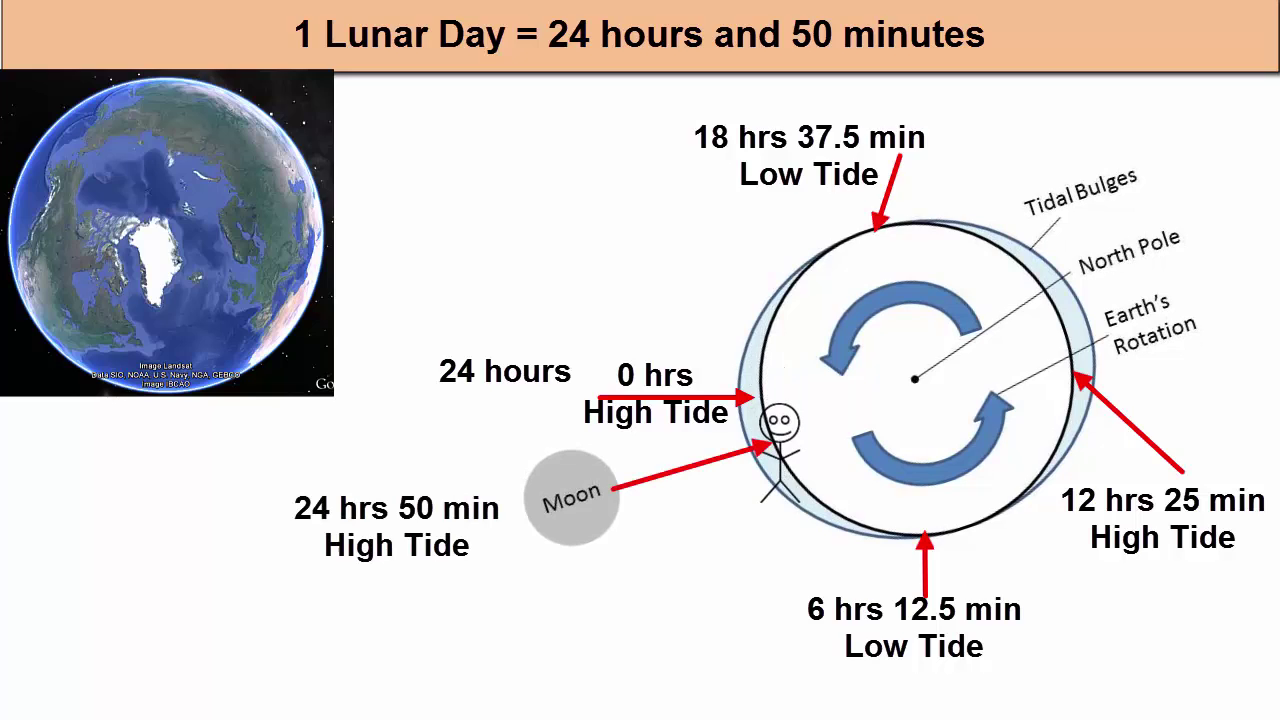
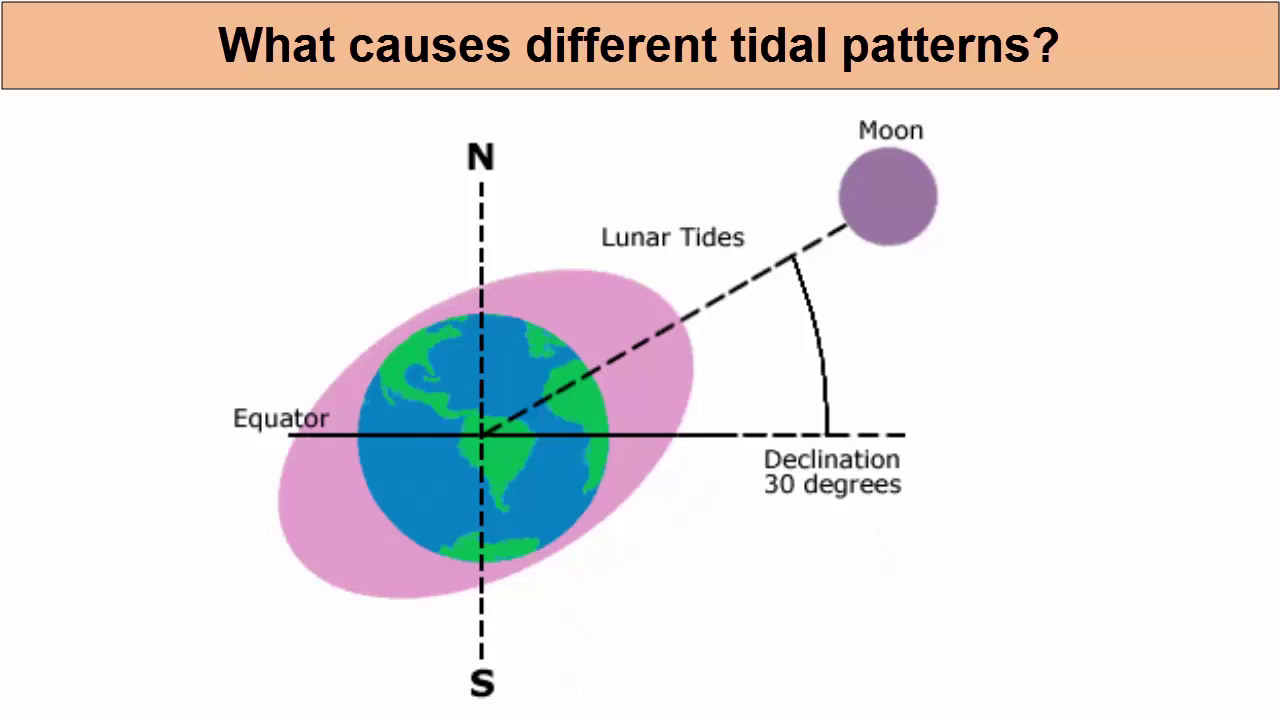
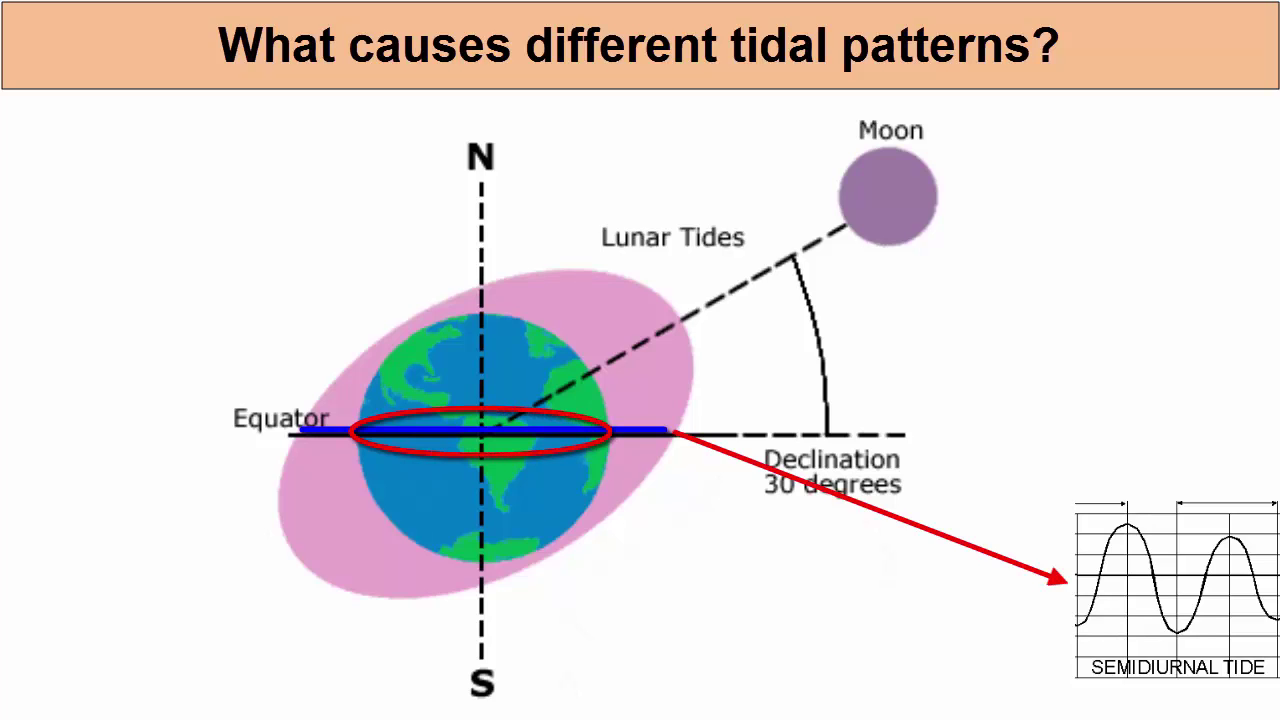
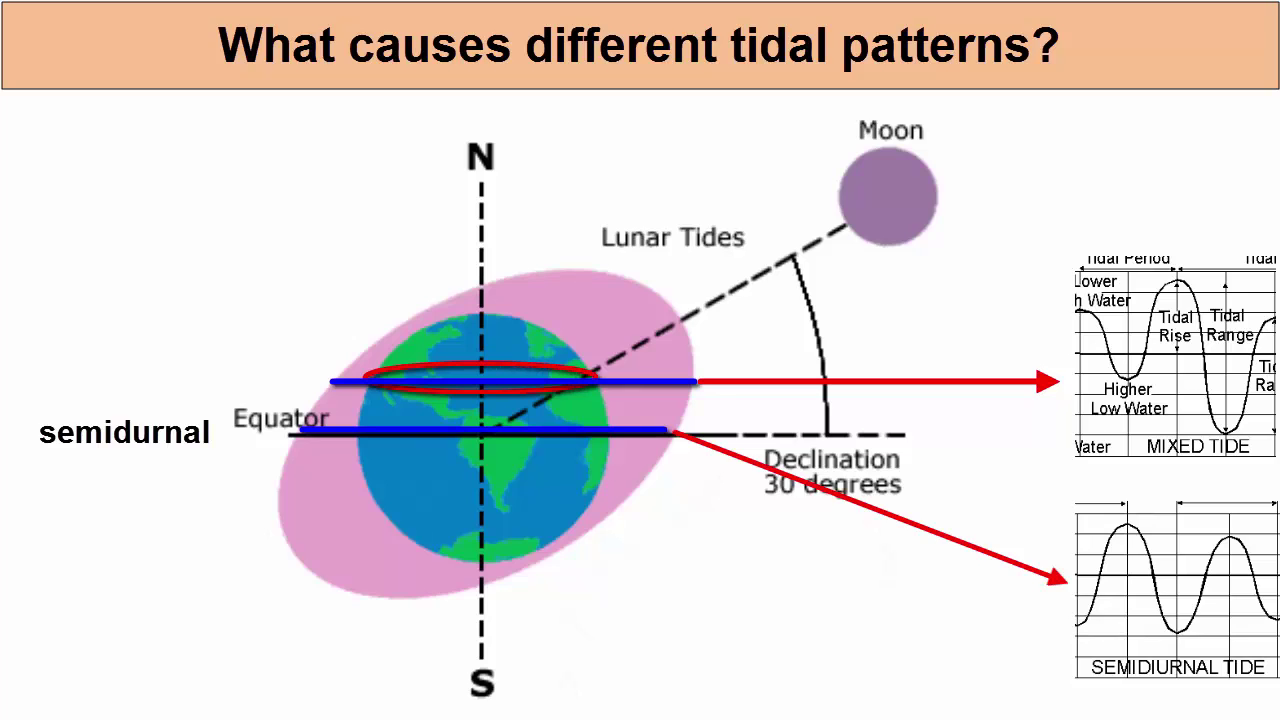
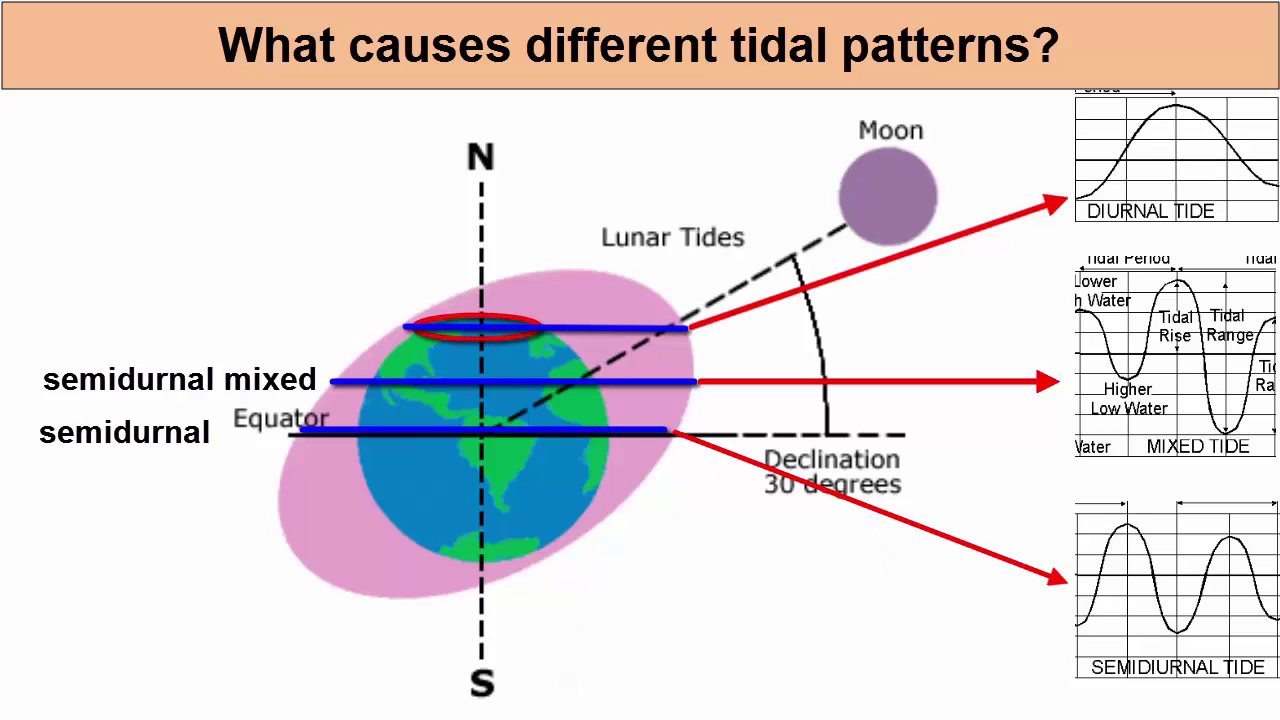
**[Segment 3: Gravitational Interactions: 2:01-2:50]** "Gravity's strength between two objects depends on their mass and distance. A simple equation involving the gravitational constant, g, explains this phenomenon. The closer the objects, or the greater their mass, the stronger the gravitational pull. This difference in gravitational force across Earth's diameter is what causes the tidal bulges."
**[Segment 4: Tidal Bulges and Earth's Rotation: 2:51-3:40]** "As Earth rotates, it passes through these tidal bulges. When we're under a bulge, we experience high tide; between bulges, it's low tide. Interestingly, the sun also influences tides. Its massive gravitational pull creates smaller tidal bulges due to its greater distance from Earth."
**[Segment 5: Tidal Interference and Phases: 3:41-4:30]** "The lunar and solar bulges interfere with each other. During full and new moons, when they align, we experience spring tides with higher highs and lower lows. In contrast, during half moons, their interference reduces the tidal range, leading to neap tides. Just by observing the moon, we can predict the tide's intensity!"
**[Outro: 6:01-6:30]** "And that concludes our journey through the tides! Next time you stand by the shore, remember the incredible celestial dance that creates the ebb and flow beneath your feet. Thanks for watching, and don't forget to like, share, and subscribe for more fascinating insights into our natural world!"
The TIdal Bulge:
The creation of the giant tidal bulge and the Earth's interaction with it is a fascinating phenomenon driven by the gravitational forces of the moon and the sun. Here's a detailed description of how the Earth creates and interacts with the tidal bulge:
1. Gravitational Forces: The moon's gravitational force pulls on the Earth, causing the water on the side of the Earth facing the moon to be pulled toward it, creating a bulge of water. Similarly, on the opposite side of the Earth, there is another bulge of water due to the centrifugal force caused by the Earth's rotation. These bulges are known as the lunar bulges.
2. Solar Influence: The sun also exerts a gravitational force on the Earth, leading to the creation of solar bulges. These bulges are caused by the sun's gravitational pull on the Earth's water, resulting in another set of bulges on the Earth's surface.
3. Interference and Tidal Patterns: The lunar and solar bulges continually interfere with each other as the moon orbits the Earth every 29 days. When the lunar and solar bulges are in phase, during full and new moons, they constructively interfere, leading to spring tides with higher high tides and lower low tides. Conversely, when the bulges are out of phase, during half moons, they destructively interfere, resulting in neap tides with lower tidal ranges.
4. Earth's Rotation: As the Earth rotates on its axis, it moves through these tidal bulges, causing the water levels to rise and fall, resulting in the ebb and flow of the tides. The Earth effectively "dances through" and "hula hoops through" these tidal bulges as it rotates. 5. Impact of Earth's Orbit: The Earth's orbit around the sun also influences the tides. The elliptical nature of the Earth's orbit means that the distance between the Earth and the sun varies throughout the year. This variation in distance affects the strength of the solar bulges, leading to variations in tidal ranges. 6. Latitude and Tidal Patterns: The Earth's tilt at a 23.
5. 5-degree angle impacts tidal patterns at different latitudes. At the equator, where the Earth rotates through two bulges of roughly the same water level, semi-diurnal tides are experienced. In mid-latitudes, the Earth moves through the two bulges at different water levels, resulting in semi-diurnal mixed tides. At the poles, only one bulge is experienced per lunar day, leading to a diurnal tidal pattern.
the creation of the giant tidal bulge is a direct result of the dyanmic interaction of the moon and the sun, leading to the formation of lunar and solar bulges on the Earth's surface. The Earth's siderial rotation and the suns orbit above the earth interact with these bulges, resulting in the ebb and flow of the tides that we know and love, or the toroidal breathing of earth.
*Waves 5.1 - Tides -
So today we're going to talk about tides. It's going to be a fairly relaxed kind of lecture. Last lecture not too many equations just descriptive. We'll talk about well tides. Tides are variations in the sea level. Right. So the first thing you have to think about is what is the sea level that you're varying around. What is the reference. Sea level. So we'll talk about the shape of the earth and the shape of the mean sea level. That's called the geoid. And then we'll look at different tidal frequencies, observations of variations in the sea level in different places. And then we'll try to explain it. So what is it that causes the tides? Anyone got any suggestions? Why do we have tides? You've all seen it. You know you've been to the beach or the Atlantic. Not so much the Mediterranean, but you go to the Atlantic coast. You see the the sea going out, coming back in because the sea level is going up and down. Why would it do that? Yeah. The gravitational you're absolutely right. It's to do with gravitational force. But it's actually quite complicated to understand. I mean, the whole Earth is subject to gravitational force, right? So why should the ocean behave differently from the rest of the planet? That's what we need to understand, right? So we'll talk about tidal forces. What are the forces responsible for these tides. And then we'll obviously the culprits are the sun and the moon. Yeah. Oh yeah. Crustal tide. Yeah. There are tidal forces which affect the Earth as well. Yeah. But very small compared to the ocean, because the ocean is a fluid which is free to move. So is the Earth. The Earth is a kind of fluid on the inside as well, but it's much more dense and much more viscous. Um, the ocean can react quickly. So that's why we have these daily variations. You see, the Earth reacts, but by the time it's reacted, the tides already. Force has already changed. You see. So we're not going to talk about that. We'll talk about the the ocean tides. The atmosphere also is subject to tidal forces. So everything has tides.
Yeah. So the sun and the moon are responsible for this tides. So we'll try to understand how and then we'll get on to more realistic, uh, detailed descriptions of how the tides vary around the world. And our old friend, the Coriolis force will come into, um, come into play. So let's just think about the shape of the Earth, then. What is a horizontal surface? A horizontal surface is a surface where if you put something on it, it won't roll one way or the other. It will stay there. If you put a ball on a horizontal surface, it'll just stay there. If the surface is not horizontal, it'll start to roll down the surface, right. So so the definition of that is something. To which the force of gravity is perpendicular. Right. It's not necessarily something which is perpendicular to a line that goes to the center of the Earth. It's something which is perpendicular to the local force of gravity. Now, the Earth is not a perfect sphere, generally speaking. Local vertical is not the same as a line that goes towards the center of the earth, and we define the horizontal surface as a surface of geopotential.
So if the force of gravity is the gradient of a geopotential, then the surface across which we have that gradient is a local horizontal surface. And so the shape of the earth, which is made up from that single surface, is called the geoid. And all departures from that geoid for the ocean surface are either associated with tides or with ocean currents. So yes, some of the some of the departures from that geoid are actually, uh, fairly steady in time because the ocean has currents. And so you have the slope of the surface to do with the local horizontal pressure gradient, which is in geostrophic balance with the force, the Coriolis force. And you have a permanent current like the Gulf Stream, so that there'll be a departure from the geoid, which is steady in time. Well, the Gulf Stream is not steady in time, but but varies over longer time scales than the daily cycle. Right. And that also needs to be taken into account. So it's variations around that which are daily which are the tides. Right. So what you need to do is look at different frequencies of variation. But before we get on to that, we just get back to this question of
what shape is the earth? So it's not a sphere, okay. It's spinning. So it's a kind of oblate spheroid, but it's actually more complicated than that because of geology and continents and all sorts of things. So this is actually the shape of the Earth. It's actually it looks a bit like a potato. This is a red presentation of the earth, which is extremely exaggerated. So all all departures from a sphere have been multiplied by some huge factor. The Earth is much more spherical than this, but this just illustrates the departure from sphericity that the Earth has. All right. And so you see this great big dip in the Indian Ocean. You see this bulge over China. You can see a bit of a dip over northern Canada. And and it's not even constant in time. This thing, it varies on geological time scales, as you know, have mountain building.
You have continental drift. That's millions of years, right.
It also varies on shorter time scales because of glacial effects. So over the last 10,000 years or so, it's gradually changed because of the disappearance of the Laurentide Ice Sheet here.
So Canada is gradually rebounding from the end of the last ice age 10,000 years ago, and it's still moving away from the center of the Earth because that weight has been lifted. Right. But for our purposes, for a daily tidal cycle, we can say this is the shape of the Earth.
So what are the variations around that? Right. So here is a time series of sea level, right. Measured at some coastal station somewhere in England. And so what do you see when you look at that that time series.
Well, the first thing you notice is that it varies quite quickly. So these are the days. This is about a month along the bottom. So within a day it's gone up and down twice basically.
And so we have two highs and two lows every day. So we have a semi-diurnal tide.
The second thing you notice is that the amplitude of this semi-diurnal tide varies throughout the month. Yeah.
So you have some periods in the month where it's quite high amplitude and some periods where the amplitude is lower.
And there are two of these maxima in the month.
If you think back to when I was talking about interference between two waves, this is quite similar to that, right? We were talking about two different wave frequencies and the packet, the modulation packet. Remember that right. So if you have two frequencies which are very similar, they can combine to make something which is a much lower frequency which modulates the amplitude of that fast signal.
This is a sign that we're looking at two different frequencies, tidal frequencies, which are both of them close to the semidiurnal frequency, but not exactly the same. So that's one way of looking at this. We'll come back to that later.
There's a simpler explanation as well. But what could it be? I mean, what what could it be that's giving you two different frequencies.
**Waves 5.2 - Tidal Forces.mp3**
So here let's let's get into trying to understand why would the gravitational force of the moon, for example, cause a semi-diurnal tide? Um, so the moon goes around the Earth. Everybody knows, right? Except that's not true. It's not entirely exactly true. Right? Um, what would happen, right, if the Earth and the moon were the same size? Would the moon go around the Earth, or would the Earth go around the moon?
Or, you know, what would happen is that they would both go around. They would kind of dance around each other, wouldn't they? They both go around their common center of mass, which would be halfway between the two. Yeah.
This is basically what's happening with the Earth and the moon. Uh, the moon does not just go around the center of the Earth, which is nailed in place. And that's very important. The Earth and the moon dance around their common center of mass.
But the Earth is something like 100 times more massive than the moon. Right. So that common center of mass is actually very close to the center of the Earth. It's actually within the Earth. It's closer to the center of the Earth than the surface of the Earth is. Right. But they are. But it's still not at the center of the Earth. And that means that as the moon goes around the Earth, the Earth is also dancing around a bit. So
. So if I'm the earth and there's the moon, the moon's going around me. And I'm also kind of doing something like that, you see. So. So if you can think about being on the earth, which is doing this kind of circuit, this small circuit, now don't confuse that with the rotation of the Earth, because the rotation of the Earth is once a day, every day. Okay. This we're talking about once a month. It's doing a circuit while it's rotating. It's also doing this little circuit okay. And associated with that alone.
Well think of think about the forces. Well, the Earth is being attracted to the moon by the moon's gravity. And also, if you want to think about some equilibrium situation, then you have to bring in this idea of the centrifugal force, because you want what we want to get at is what is the effect of a fluid on the earth. Right. So it'll be also subject to this centrifugal force, which is uh, has a tendency to be drawn away from the moon relative to the rest of the Earth.
Okay. So that's centrifugal force is pushing it. It's the same everywhere because its rotation is the same everywhere, and it's pushing it away from the moon. So then we can talk about some equilibrium between the attraction, the gravitational attraction of the moon, which is attracting the Earth, and also the ocean, right. And the centrifugal force which is pushing, pushing the ocean in the opposite direction to the moon. Okay. And so this is where, you know, this is where this the subtlety lies. Okay. So that gravitational force is here's the formula.
It's big G, the universal gravitational constant which is this. It's 6.672 times ten to the -11 newtons meter squared per kilogram squared times the mass of the Earth, times the mass of the moon divided by the distance between their centers squared. Okay. That is the law of gravity, right?
So that's the the gravitational force between the Earth and the moon. Right. What about this centrifugal force? Well, it's going to be the same at the center of the earth. It's going to be the same because they're in balance. Right? So if we think about the net force that's generating the tide, then at the Earth's center, here's the blue arrow. Is gravity the force of gravity attracting us to the moon. The red arrow is the centrifugal force which is equal and opposite at the Earth's center.
Right. But here's the thing. Right closer to the moon, there'll be a slightly stronger gravitational attraction because you're closer. Right. And it's one over r squared law here. So at this point here, for example, the net force centrifugal force won't change in that direction. That's this tum. Right. But the force in this direction will be slightly stronger. On the denominator you'll have r minus a squared where a is the radius of the earth. So gravity wins here.
And you have a net force towards the moon on the opposite side of the earth. The net force is away from the moon because the centrifugal force is the same, but gravity is a bit weaker. You have an R plus A here, right? So there's a stretching effect on this. On the side facing the moon. The ocean is attracted to the moon. On the side opposite the moon the ocean is pushed in the opposite direction to the moon. So this kind of stretching effect. And then if you look at the other latitudes, you see the Earth is not that far away from the moon. That's why this is important. How far away is the moon? Anybody know? It's 380,000km, right? It's it's not that far. It's a three day trip in a spaceship. Right? If you get away from the Earth, it takes you. The difficult thing is to get away from the Earth, right? Once you've done that, three days later, you're at the moon. You could even drive there. I mean, I don't know, 380,000km. It's a bit of an old car, but it's. There are cars that have done more than that, right? It's within our kind of personal understanding. Most of us probably drive that distance in our lifetimes quite easily. So it's within our kind of intuitive grasp, the distance to the moon. And it's close enough that these forces, right, are not even parallel. There's a certain tilt here. It's exaggerated here, obviously. So then if you look at the resultant, it will have a horizontal component.
That is actually also very important because this force here, even though it's lifting the ocean, it's got a huge job to do. It can't compete with the gravity of the Earth. It's tiny compared to the Earth's own gravity. Right. So that wouldn't actually make a tide. But the fact that you have these converging horizontal components, that's what makes the tides possible, because they can allow the ocean to flow in sideways and create this bulge. Okay. So the horizontal forces and the vertical forces are important in creating what we're going to see is a kind of tidal bulge okay. So that's the next thing.
So in summary what have we got here.
We've got a difference between two forces. Because the Earth is a finite object in space which is not too far from the moon. The radius of the Earth is 6400km. The distance to the moon is 380,000km on average. And so these two terms are different enough to have an effect. You can combine those two terms to write the equation in full here and then simplify it. And it's approximately equal to this g times n one times m two times two times the radius of the earth divided by r cubed. So that's important. You get an R cubed on the bottom.
So the actual gravitational force between the Earth and the moon. That's a one over r squared law. But the force that generates the tides that's a one over r cubed law, which means that if you're closer to the object, it's going to have a much more a much stronger effect. And that's why the moon is so important. Right? The sun is important as well. Right?
The sun generates tides, but the moon actually has a stronger tidal effect than the sun. Not because the gravitational force is stronger, it's actually a lot weaker. Gravitational force from the sun is massive. Much stronger than the force from the moon. Right. But this tide generating force, because the moon is so much closer than than the sun. The moon is 380,000km.
The sun is 150,000,000km. That means that the moon actually wins. It's about twice as important as the sun for generating tides. Right? So what's the effect of this tide generating force? So you've got here's the moon, here's the Earth. Um, and all over the Earth you'll have these you can draw these force arrows. So let's suppose that these force arrows will then displace the ocean into a new equilibrium shape. And it'll be this kind of oval shape.
There'll be a lobe which is pointing towards the moon, and another lobe which is pointing away from the moon. Right. If you like fruit, okay. You can use the fruit analogy. It's like an orange inside a melon. Okay. So you've got a spherical object inside a prolate spheroid. Or if you like sport, you can use a sports analogy. It's like a football inside a rugby ball okay. And yeah. So if there's any Americans watching a football is a spherical object okay. Which you kick with your feet. And that's why the game is called football. All right. Imagine a football inside the long pointy thing that's called a rugby ball. Football inside a rugby ball. But the football is spinning okay. So if, um, you go round the back and then here and then round the front again, imagine that you're spinning inside this prolate spheroid. You will encounter a deeper ocean here. Okay. And then around the back you'll have a shallower ocean like that okay. But here round the back. And then on the other side, half a day later, you'll have a deep ocean again. And then you come round the front and it'll be shallow again, that kind of depth. And so there you go. Two tides per day. All right. That's the semi-diurnal tide. You just have to imagine the this football rotating inside this fixed rugby ball.
**Waves 5.3 - Tidal Periods and Amplitudes.mp3**
That's the semi-diurnal tide. You just have to imagine the this football rotating inside this fixed rugby ball. Except obviously it's a bit more complicated than that because the moon doesn't just stay where it is, does it? Um, so what is the actual tidal period? The Earth rotates within the bulge, producing two tides per day. So here we have that sort of plan view of that. So here's the North Pole. The Earth's rotating like this in these four positions 1234. So high tide, low tide, high tide, low tide okay. This is the exaggerated tidal bulge. But by the time it gets back here again, well, the moon's gone. It's not there anymore. I mean, you've noticed. I mean, I'm sure you must have noticed that, um. The moon doesn't rise at the same time every night, right? Full moon. The moon rises about the same time as the sun sets. Yeah, because it's a full moon. That's kind of obvious, right? The next day it doesn't rise at the same time. It rises about an hour later. Right. So you think, oh, I'm going to watch the moon rise again, and then you're hanging around for an hour thinking, what's it doing? Where is it? And and then it finally comes up. Right. And you've experienced that. It's because the moon is orbiting the Earth, right. So so the Earth goes round. So the Earth starts here X it goes around. That's one day, okay. That's one sidereal day. And then you've got to the moon's buggered off over here. So you've got a carry on a bit. Another 50 minutes. Well a bit more than that actually. Um because so the actual period is 24 hours and 50 minutes. Right. So. So this sidereal day here is actually, uh, 23 hours and 56 minutes, because at the same time, the Earth is going around the sun. The solar day is 24 hours. So anyway, this tidal period here is 24 hours and 50 minutes. So half of that is 12 hours and 25 minutes. That's the period of the lunar semi-diurnal tide, 12 hours and 25 minutes. But actually it's not that simple either, because up to now we've assumed that everything's in equilibrium. Right? So the moon, wherever the moon is, that tidal tidal bulge is pointing at the moon. All the forces are balanced all the time. Right now, that is a fundamental assumption of tidal theory. Well, is it true? Does it work? Is it actually possible for this bulge to go around the Earth, for the Earth to rotate under this bulge would imply that this bulge is traveling around the Earth, right. Is it possible for a tidal wave to travel around the Earth at that speed? How fast can a wave go at the equator? For example, how fast can a wave go and how fast does it need to go? We can work it out. Let's do it on the blackboard. Right. So here's the earth and it's going around. How fast is it going at the equator? That's the first question.
Um, so here's a North Pole, right? So what's the. Well, it's speed is is distance divided by time. So it's two pi times the radius of the Earth divided by 24 times 30, 600. Okay. Um, uh, where a equals 6.4 times ten to the six meters. Right? 6400km. Someone to be somebody. Work that out for me. What's this? Speed. Two pi times that. Divided by that. That will be in meters per second. So we're thinking about a wave. A tidal wave, right. Which has to travel at that speed. Well, it has to basically go the other way, doesn't it. Relative to the Earth, relative to the rotating Earth. Come here. 460 465. Is that what you said? Yeah. Sorry, I didn't hear you. 465.
Meters per second. Is that possible? Can a wave go that fast? How fast does the wave go? It's a shallow water wave because it's very large scale. The ocean is five kilometers deep. So we've got the phase speed is equal to root root g h okay. Which is equal to the square root of 9.81 times. What's the depth of the ocean. Five kilometers okay. So 5000. Somebody do that for me. Yeah 221 221m/s. All right. So compared to 465m/s, it's not going to keep up is it. At the equator. So um, we're talking about the Earth rotating inside this bulge. And this bulge is pointing to the moon. This bulge can't keep up with the moon. So it's actually a bit more complicated than just saying it's an equilibrium tide. But the forcing frequency doesn't change. The forcing frequency is still 12 hours and 25 minutes, right? So it won't keep up, but a day later it'll still be attracted to the same position. It'll just do some non equilibrium thing which will have a period of 12 hours and 25 minutes. Is it that simple though? That's the question. And no actually it's a little bit more complicated than that because the moon is not in the equatorial plane right. Neither is the sun, right? The angle between the moon and the equatorial plane is 28.5 degrees, so it's quite a quite a big angle compared to the sun. I mean, the sun is 23.5 degrees, so which means that the the plane of the moon moon's orbit is five degrees off from the plane of the Earth's orbit around the sun. Anyway, so 28.5 degrees tilted compared to the equator, which means that this bulge here is not lined up perpendicular to the axis of the rotation of the Earth. Right? So if you imagine now that the bulge is pointing at the moon at this 28.5 degree angle, what would that high tide look like? Well, here's a high tide here. And then the other high tide on the other side. If you look at this latitude, well, it's not so high, is it. Because this is directly pointing with the bulge. But the bulge, the other end of the bulge is down in the opposite hemisphere here. So at the same latitude, the same position on the Earth, you have one big high tide and one small high tide. And then in between the two, the low tides are still the lowest you can get. Like this. An extreme example of that would be at a slightly higher latitude. The big high tide would be no bigger than the low tide. And so what you'd end up with is just one tide per day. So you can have combinations of signals from a diurnal tide and a semi-diurnal tide. That's the way it's usually described. Just because of this tilt in the plane of the moon's orbit compared to the equator. There are other reasons why you have different signals semidiurnal and diurnal, which we'll get on to later, which if you want to in a more realistic context. But there's a simple example of how even with a spherical earth completely covered in ocean, you will still have a combination of a diurnal tide and a semi-diurnal tide. If the orbit of the moon is slightly off, let's add another complication the orbit of the moon is not circular, right? You have this elliptical orbit, right? Which means that sometimes the moon is closer and sometimes it's further away. Now, as we said at the beginning, the closer you are, the stronger the tides, which means the amplitude of the tide varies throughout the month, because when the moon is closer, you have a stronger tide, and when it's further away, you have a weaker tide. If you happen to have a full moon when the moon is close, you call that a supermoon, right? And people talk about that you see on the news like, oh my God, there's going to be a supermoon. As if it was really rare. You have at least one every year, right? Because when the moon is opposite the sun, that's a full moon and this is the lips is going to arrange itself like that at least once a year. Right? Except this ellipse is not actually fixed in space. It has an 18.6 year precession period as well. So there's another time scale, uh, a very long time scale of variation of the tides. 18.6 years. Right. See, more and more time scales coming into this now, like there's a 13% difference in the distance to the moon. That's going to change the period of the tides as well. So here we have to use, uh, Kepler's law of equal areas. If you have, um, here's the Earth, right. And you have an elliptical orbit.
Right. And so the moon is the moon going around the Earth. It will sweep out equal areas in equal times.
All right.
So it's going faster when it's closer so that it can sweep out the same area slower when it's further away. Right. So that will change the tidal period. It won't always be 12 hours 25 minutes. Sometimes it'll be a bit more. Sometimes it would be a bit less. So what's it going to be here? Is it going to be more than 12 hours, 25 minutes or less? Who says more? Hands up for more. Who says less?
You're all wrong.
It's going to be more so it takes longer. The moon moves further right in a day, for example. Right? Which means that the Earth takes longer to catch up with it again. So the tidal period will be slower, right? And here the moon's going slowly, so it'll be much closer to 12 hours because the Earth catches up with it sooner. I'm going to say stronger tides and longer period. And then we have weaker tides and shorter period here. Right?
**Waves 5.4 - Lunar and Solar Tides.mp3**
So that's the moon. Time to bring the other player into the picture. And that is the sun. Now the earth goes around the sun, right? And the sun causes tides as well. And so here's the earth going around the sun. And that orbit is not perfectly circular either, right? It's elliptical, but the eccentricity of that ellipses is somewhat smaller.
's only a 4% difference between the closest and the furthest away. And as I said before, the axis of the Earth's rotation is tilted compared to the plane of the orbit around the sun. And that's what gives us the seasons we have.
The winter solstice is the 21st of December, right? And then the equinox, when the days and the nights are the same length 21st of March and then the summer solstice, 21st of June or thereabouts. It varies, sometimes from one day to another. So yeah, this 4% difference between perihelion and aphelion will have a small effect on the solar tide.
But what is the period of the solar tide? We established that the lunar tide would be 12 hours and 25 minutes. What about the solar tide? It's actually pretty simple because. It's half a day, right? A day is defined as the time between when the sun's in the middle of the sky, and then when it comes back around to the same position, which is 24 hours.
And that's what we're interested in for this gravitational bulge which points towards the sun. Right.
So the solar semidiurnal tide will be exactly 12 hours. Or thereabouts. Okay. Because again, we have this Kepler effect, right? Which nobody talks about. You have your watches are set so that they will always run a day for 24 hours. And that's what you call Greenwich Mean time. Okay. Anyone ever think about what GMT actually means?
Greenwich Mean Time? The M there stands for mean and it's because in reality, the days are not all the same length, the days are not all 24 hours. And we just ignore that because it would be very inconvenient.
The mean there is because of that, because of this Kepler effect and how sometimes the Earth is moving fast around the sun than at other times. And when it's moving fast around the sun, the actual day is a bit longer because it takes a bit longer to get back facing the sun again. Right? So when we're closer to the sun around a 3rd of January, the days are actually longer. I'm not talking about the the the sunlight time. I'm talking about the entire 24 hour day.
It's actually more than 24 hours. And then when we're further away, it's less than 24 hours. So that will affect the tidal frequency for the solar tide. Now let's put the two together. And so we have these two different frequencies. I showed you the interference between those two frequencies 12 hours and 12 hours, 25 minutes that gave you this modulation. So twice a month you have these strong tides and then twice a month you have the weaker tides.
There's a simple way of understanding that you can just say, look, here's the sun, here's the moon, and here's the earth. And the light blue bulge is the lunar tide. And the darker blue bulge is the solar tide. And in this configuration with the new moon, they are reinforcing one another. So they add together and you get a strong tide. It's called a spring tide.
A week later the solar tide is in this orientation in the lunar tide. Here's the moon. It's a growing half moon. They are canceling one another out to some extent. Now, as I said, the lunar tide is stronger than the solar tide.
So you won't get you won't get zero tide here because the lunar tide will always win. It's about twice as big as the solar tide, but you'll get a weaker tide because they're canceling. And then full moon a week later, they're reinforcing one another again.
So there you go.
Twice a month you get these strong tides, and then twice a month you get the weak tides. So what do we mean when when we say a month, what exactly do we mean? Well, here's the actual orbit of the moon around the Earth.
It just jumps like that as the Earth goes around the sun and you see the Earth wobbles a little bit as it as it goes around as well. I think that's exaggerated, but. So you've got this.
And so how long does it take for the Earth to move that distance of one moon orbit? Well, the moon goes around the Earth in 27.3 days, right? But in the meantime, the Earth has moved. So relative to the sun, the moon has to go another couple of days to 29.5 days to be in the same phase again. So the phases of the moon change on a period which is about 30 days, 29.5 days. So the time between a new moon and a full moon, the time between these maxima in tidal amplitude is about 15 days.
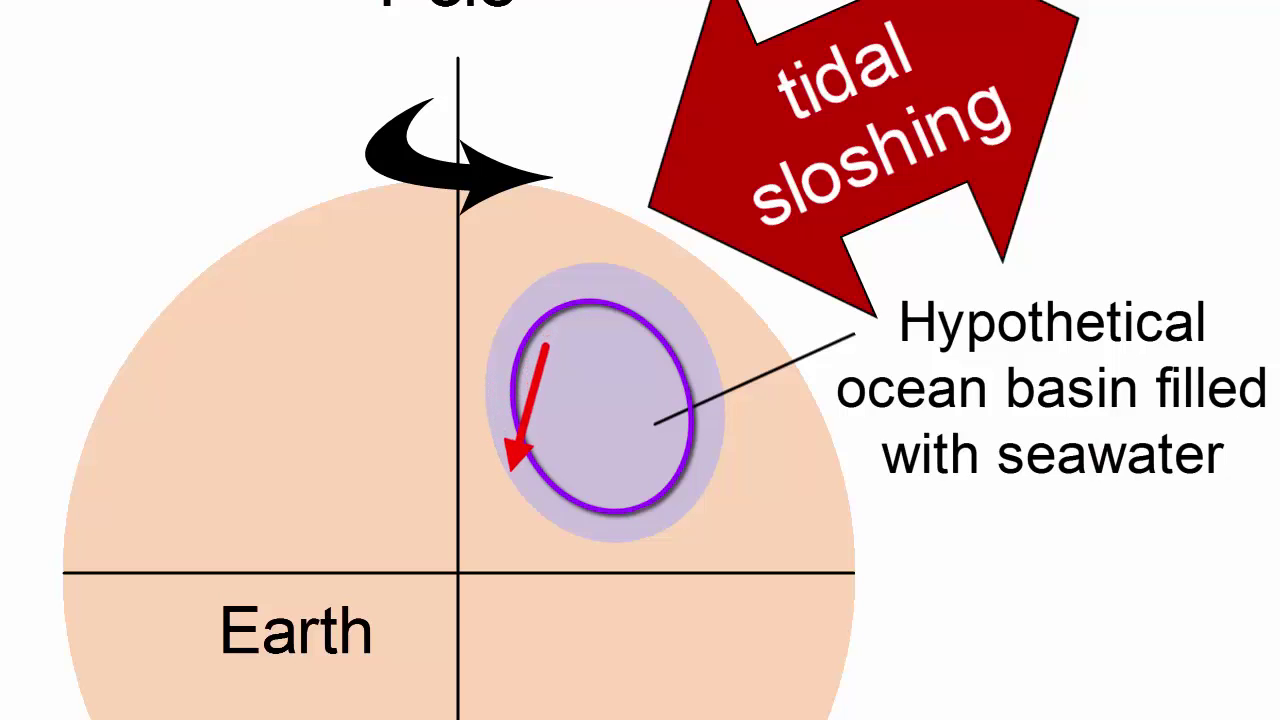
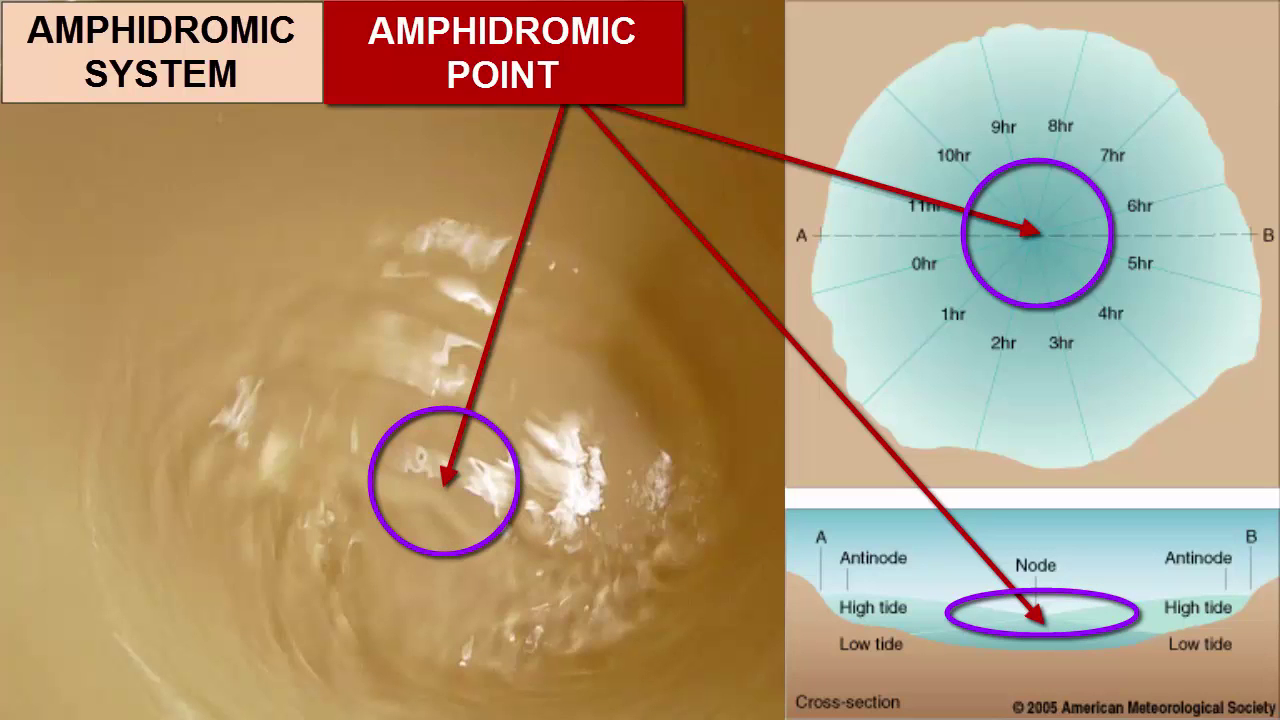
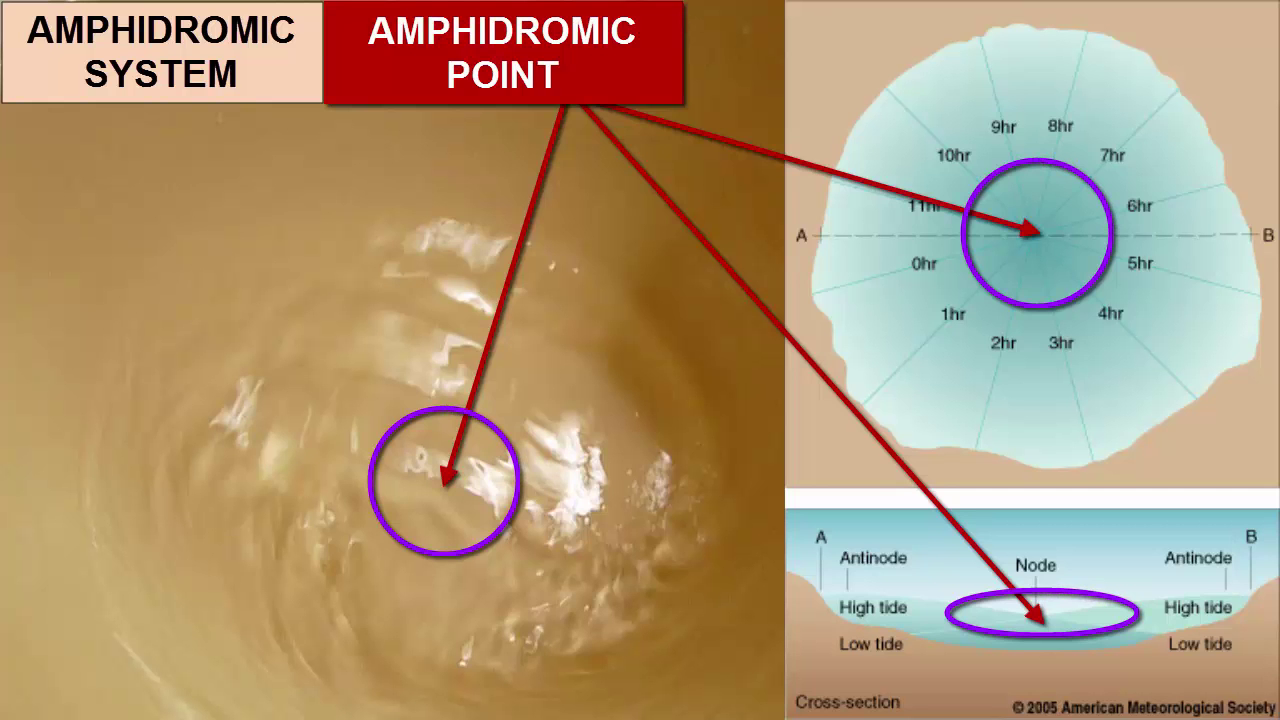
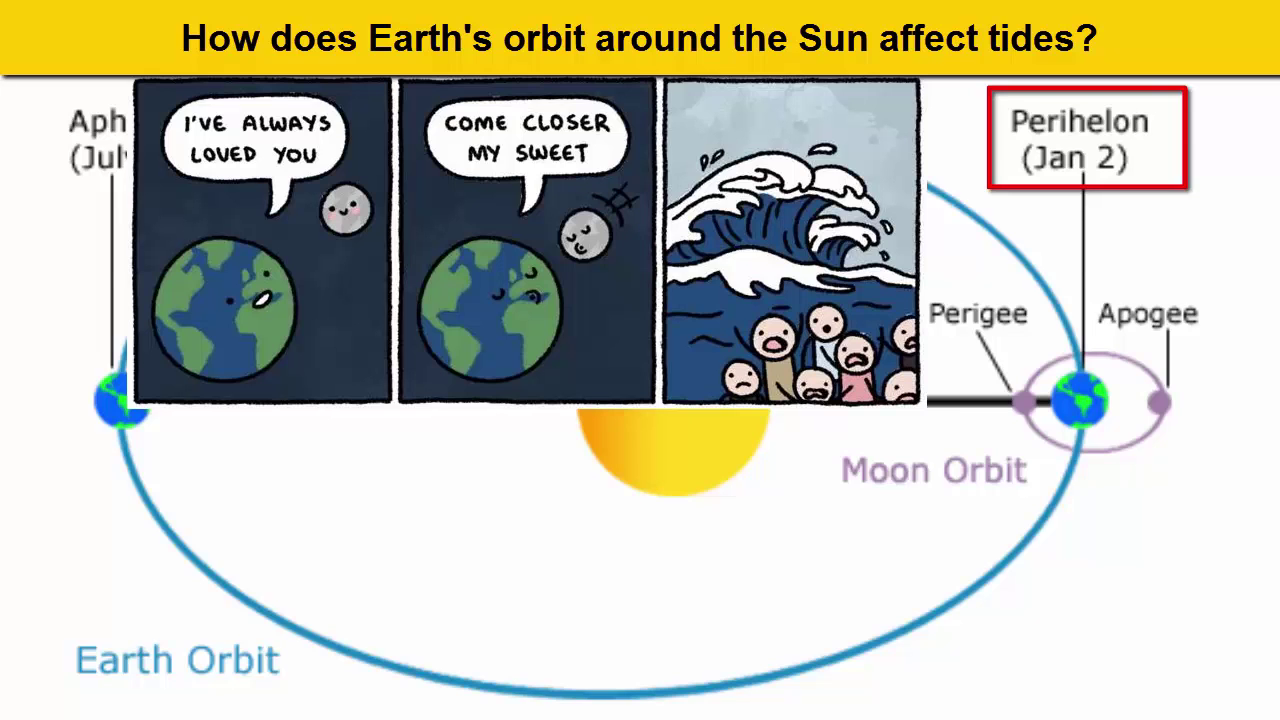
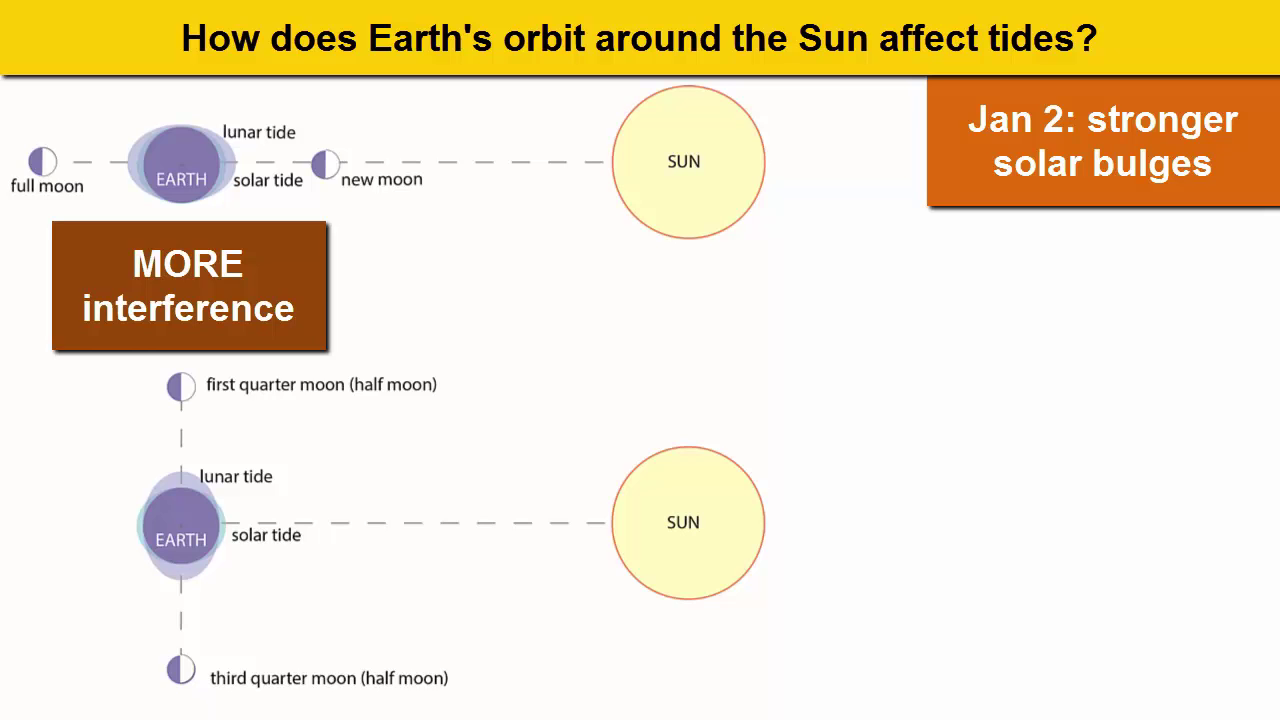
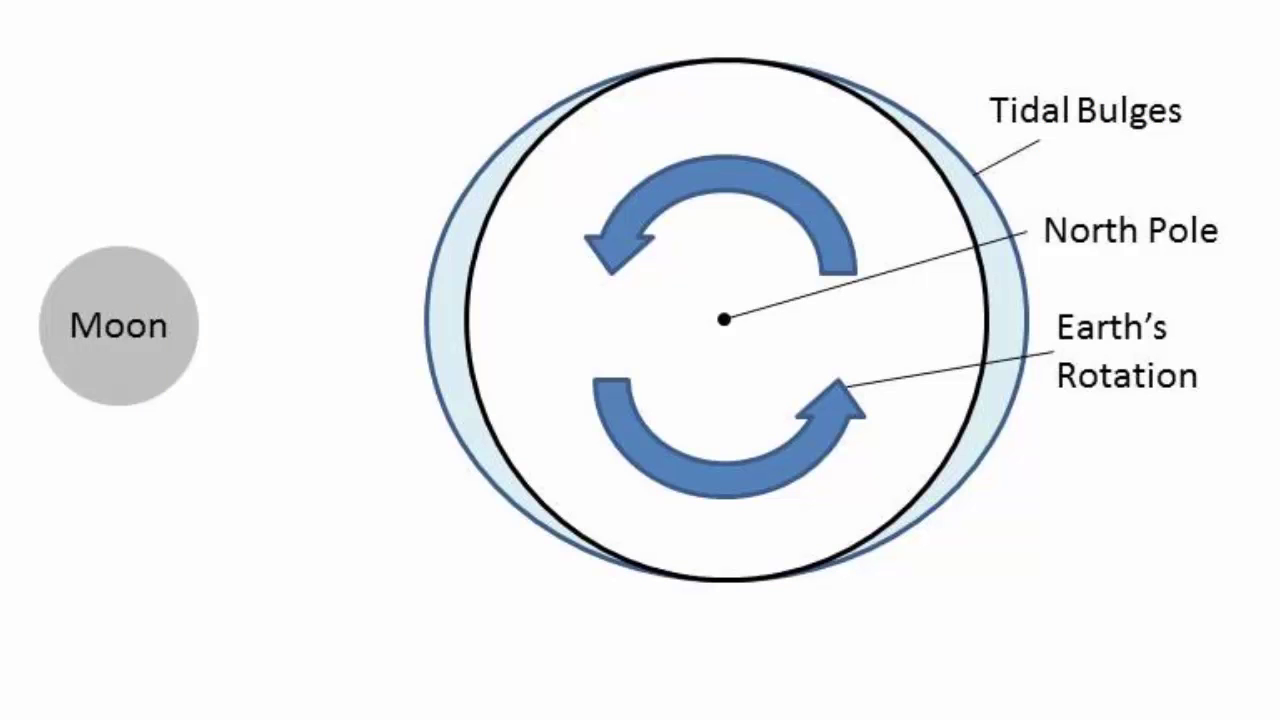
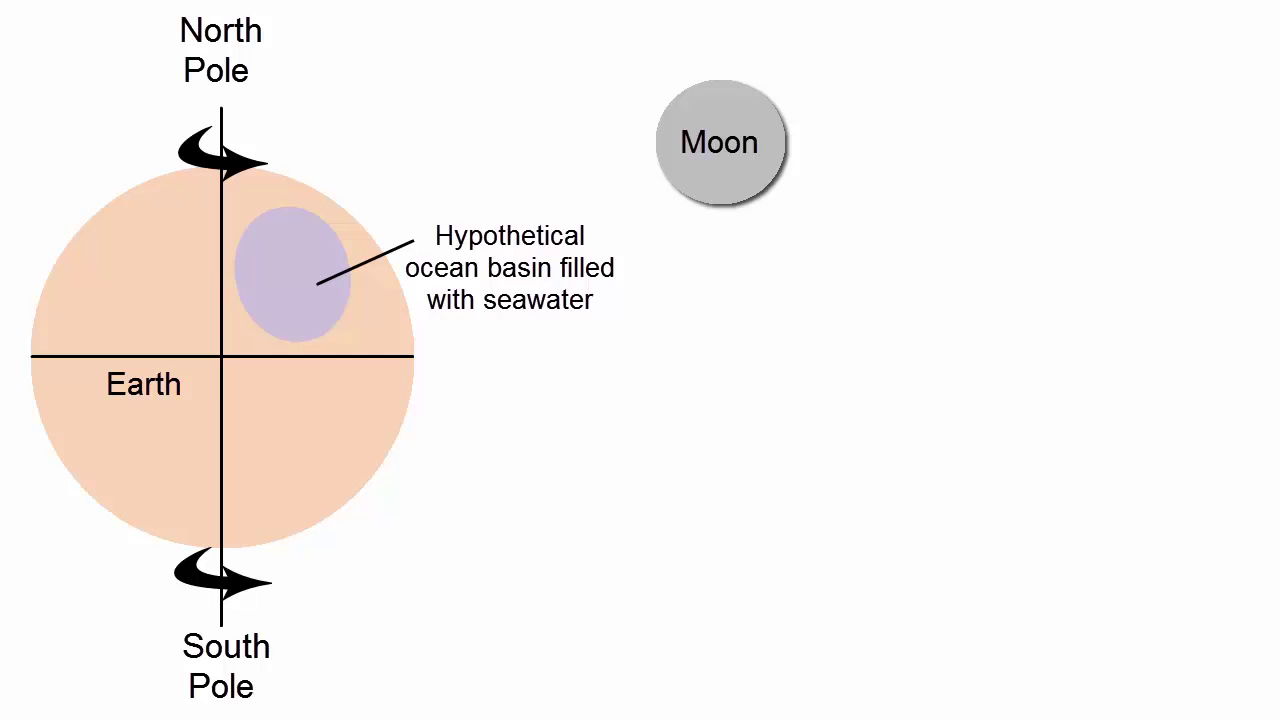
**Waves 5.5 - Tides in Ocean Basins.mp3**
So we're going to move away from the idea that the Earth is just a ball covered with ocean now, and look at real oceans with real coastlines. And this is the kind of map that you draw. So the blue lines are amplitude and the red lines are phase, right. And what we have is maximum amplitudes are close to the coasts generally speaking. And then you do have these points where the amplitude is zero. Now this map has been drawn for these semidiurnal lunar component right. The M2 tide. You have to draw a different map for every component of the tide. So you look at the variations of this exact frequency and this is the pattern that you get. So maximum amplitudes are near the coasts. There are regions where the amplitude is zero where these red phase lines come together. Right. So here in the Mid-Atlantic North Atlantic here there's no tide. Here on the coast you have the maximum amplitude. And it it will obviously vary during the day on a, on a 12 hour 25 minute period. And so that maximum will will be seen at different times of day. So here's zero two hours later. Four hours later, five, six, seven, eight all the way around to 12 hours. Um, it propagates around, okay. And you can see it's going around anticlockwise around this nodal point. So this is called the Amphidromic dynamic system. And this is a amphidromic node here. And so you have the tide circulating around that node on the tidal period with its maximum amplitude usually near near the coast. Here's another one. Um going around this way. Here you've got one, two, three, four also going around this way. Um, here's one where it goes around the other way. Right. Um, here as well. It's going around clockwise. So where is it going? Around anticlockwise. And where is it going around clockwise. Well, it depends on the exact shape of the coasts and the arrangements of the ocean basins, but a simple minded explanation would be that in the Northern Hemisphere it goes anticlockwise, and in the southern Hemisphere it goes clockwise. There are exceptions to that, though. So why would that be? Why would the phase propagate around a nodal point. Anticlockwise here but clockwise here. Right. Well let's just stick with the northern hemisphere. Why is it going around anticlockwise? Does it remind you of anything? Does it remind you of anything from from the course which we when we were talking about wave dynamics, we studied a wave called a Kelvin wave, didn't we? Which is trapped on the coast.
Huh?
And we said that in the northern Hemisphere, that Kelvin wave propagates with the coast to the right. And that's exactly what's happening here. The tide propagates around the coast as a Kelvin wave. It's just a gravity wave propagating around a coast. It's a gravity wave that has been forced by gravitational force. It's propagating around the coast. It is a Kelvin wave. Right. So here's another way to explain why a Kelvin wave propagates in that way. Let's imagine that we're forcing the water into this closed basin here. Right. So the water comes rushing in and as it moves into the basin, because it's been attracted there by the, by the moon, right? It experiences the Coriolis force which pushes it to the right. So it piles up on the right hand coast here. Right. And then six hours later, it gets pulled out again by the tidal attraction. Right. And as it comes out again, it's pushed to the right by the Coriolis force. So it'll pile up against the opposite wall. So in the process of a tidal cycle, it has gone around the basin like that, okay. Around these phase lines like that anti-clockwise. Just like a carbon wave. Because that's what it is. Now here are a few examples of tides of different frequencies we have. The thing we saw at the beginning comes from somewhere in England where there's a very clear semi-diurnal tide. Right. So we have two tides per day and we have modulation with a with every 15 days we have a peak and the tidal amplitude. Right. Here's San Francisco. Now there you have. Sometimes it looks like there's semidiurnal here for example. Sometimes it looks a bit more diurnal. So there's obviously a mixture of the two frequencies going on here, but it's mostly semidiurnal. Right. Then we go to Manila in the Philippines and it's another mixture. But the diurnal frequency is dominating here. You've got a few semidiurnal signature days where the tidal amplitude is a bit lower here, but when you have the high tides, it's diurnal. And then Vietnam, um, in Vietnam you have a very clear diurnal tide, not necessarily for the reasons I was explaining earlier, just to do with latitude. It's also to do with the size of the basin, how long it takes a Kelvin wave to go around. That's what picks out these different frequencies, right? Traditionally, tidal science is just a question of analyzing a whole bunch of different frequencies. Right. And they give them names. Right. So the lunar semidiurnal tide is called the M2 tide. The Solis semidiurnal tide is called the S2 tide. That sort of makes sense. And then there's it gets a bit more, um, complicated after that. But basically we have the period of the, for example, the solar semi-diurnal tide, 12 hours. That's the average period, right? The moon, that's 12.42 hours. So 12 hours and 25 minutes. The amplitude, um, well, we call it 100 for the moon. That's just by definition. And then the sun it's it's 46.6. So it's about half as strong as the lunar tide. And then you have all these other components which have different amplitudes, different periods, um, semi-diurnal diurnal and longer periods. And they all have letters and names and, and tidal models take into account all these components. I'm going to show you a couple of components. So here are maps of amplitude around Europe. Um in the North Atlantic, here of the M2 tide, the S2 tide, a couple of other semidiurnal components. And what do you reckon? Um, so lunar tide, solar tide, um, they're all semidiurnal and they all look the same, right? They all look fairly similar. It's not surprising, because what we have here is the response of a dynamical system to forcing at a particular frequency. Right. So the system doesn't care, uh, which astronomical object is doing the forcing. Right. It's just going to respond in the same way. But then if you look at the diurnal tide, it looks different. They all look the same as well, but they look different to these ones. Right. So there you go. That's the amplitude pattern for the for the tides. And if you will go back to this picture that I showed you when I was talking about Kelvin waves, um, if you look at the tidal range, southern England, it's just a few meters northern France it's up to ten meters. Right. So the tide is stronger in northern France than it is in southern England, because it's a Kelvin wave propagating with France to its right as it goes through the English Channel. Right. And here's a picture of that power station in, in Saint-Malo, where they generate energy from the tidal.
Um.
Daily variations. Okay. And I think that that is the end of the course. So I think that if you if you like the course, then everybody should give me like a, like a thumbs up. Right. All right. Give me a thumbs up, everybody.
All right.
The TIdal Bulge:
The creation of the giant tidal bulge and the Earth's interaction with it is a fascinating phenomenon driven by the gravitational forces of the moon and the sun. Here's a detailed description of how the Earth creates and interacts with the tidal bulge:
1. Gravitational Forces: The moon's gravitational force pulls on the Earth, causing the water on the side of the Earth facing the moon to be pulled toward it, creating a bulge of water. Similarly, on the opposite side of the Earth, there is another bulge of water due to the centrifugal force caused by the Earth's rotation. These bulges are known as the lunar bulges.
2. Solar Influence: The sun also exerts a gravitational force on the Earth, leading to the creation of solar bulges. These bulges are caused by the sun's gravitational pull on the Earth's water, resulting in another set of bulges on the Earth's surface.
3. Interference and Tidal Patterns: The lunar and solar bulges continually interfere with each other as the moon orbits the Earth every 29 days. When the lunar and solar bulges are in phase, during full and new moons, they constructively interfere, leading to spring tides with higher high tides and lower low tides. Conversely, when the bulges are out of phase, during half moons, they destructively interfere, resulting in neap tides with lower tidal ranges.
4. Earth's Rotation: As the Earth rotates on its axis, it moves through these tidal bulges, causing the water levels to rise and fall, resulting in the ebb and flow of the tides. The Earth effectively "dances through" and "hula hoops through" these tidal bulges as it rotates. 5. Impact of Earth's Orbit: The Earth's orbit around the sun also influences the tides. The elliptical nature of the Earth's orbit means that the distance between the Earth and the sun varies throughout the year. This variation in distance affects the strength of the solar bulges, leading to variations in tidal ranges. 6. Latitude and Tidal Patterns: The Earth's tilt at a 23.
5. 5-degree angle impacts tidal patterns at different latitudes. At the equator, where the Earth rotates through two bulges of roughly the same water level, semi-diurnal tides are experienced. In mid-latitudes, the Earth moves through the two bulges at different water levels, resulting in semi-diurnal mixed tides. At the poles, only one bulge is experienced per lunar day, leading to a diurnal tidal pattern.
So today we're going to talk about tides. It's going to be a fairly relaxed kind of lecture. Last lecture not too many equations just descriptive. We'll talk about well tides. Tides are variations in the sea level. Right. So the first thing you have to think about is what is the sea level that you're varying around. What is the reference. Sea level. So we'll talk about the shape of the earth and the shape of the mean sea level. That's called the geoid. And then we'll look at different tidal frequencies, observations of variations in the sea level in different places. And then we'll try to explain it. So what is it that causes the tides? Anyone got any suggestions? Why do we have tides? You've all seen it. You know you've been to the beach or the Atlantic. Not so much the Mediterranean, but you go to the Atlantic coast. You see the the sea going out, coming back in because the sea level is going up and down. Why would it do that? Yeah. The gravitational you're absolutely right. It's to do with gravitational force. But it's actually quite complicated to understand. I mean, the whole Earth is subject to gravitational force, right? So why should the ocean behave differently from the rest of the planet? That's what we need to understand, right? So we'll talk about tidal forces. What are the forces responsible for these tides. And then we'll obviously the culprits are the sun and the moon. Yeah. Oh yeah. Crustal tide. Yeah. There are tidal forces which affect the Earth as well. Yeah. But very small compared to the ocean, because the ocean is a fluid which is free to move. So is the Earth. The Earth is a kind of fluid on the inside as well, but it's much more dense and much more viscous. Um, the ocean can react quickly. So that's why we have these daily variations. You see, the Earth reacts, but by the time it's reacted, the tides already. Force has already changed. You see. So we're not going to talk about that. We'll talk about the the ocean tides. The atmosphere also is subject to tidal forces. So everything has tides. Yeah. So the sun and the moon are responsible for this tides. So we'll try to understand how and then we'll get on to more realistic, uh, detailed descriptions of how the tides vary around the world. And our old friend, the Coriolis force will come into, um, come into play. So let's just think about the shape of the Earth, then. What is a horizontal surface? A horizontal surface is a surface where if you put something on it, it won't roll one way or the other. It will stay there. If you put a ball on a horizontal surface, it'll just stay there. If the surface is not horizontal, it'll start to roll down the surface, right. So so the definition of that is something. To which the force of gravity is perpendicular. Right. It's not necessarily something which is perpendicular to a line that goes to the center of the Earth. It's something which is perpendicular to the local force of gravity. Now, the Earth is not a perfect sphere, generally speaking. Local vertical is not the same as a line that goes towards the center of the earth, and we define the horizontal surface as a surface of geopotential. So if the force of gravity is the gradient of a geopotential, then the surface across which we have that gradient is a local horizontal surface. And so the shape of the earth, which is made up from that single surface, is called the geoid. And all departures from that geoid for the ocean surface are either associated with tides or with ocean currents. So yes, some of the some of the departures from that geoid are actually, uh, fairly steady in time because the ocean has currents. And so you have the slope of the surface to do with the local horizontal pressure gradient, which is in geostrophic balance with the force, the Coriolis force. And you have a permanent current like the Gulf Stream, so that there'll be a departure from the geoid, which is steady in time. Well, the Gulf Stream is not steady in time, but but varies over longer time scales than the daily cycle. Right. And that also needs to be taken into account. So it's variations around that which are daily which are the tides. Right. So what you need to do is look at different frequencies of variation. But before we get on to that, we just get back to this question of what shape is the earth? So it's not a sphere, okay. It's spinning. So it's a kind of oblate spheroid, but it's actually more complicated than that because of geology and continents and all sorts of things. So this is actually the shape of the Earth. It's actually it looks a bit like a potato. This is a red presentation of the earth, which is extremely exaggerated. So all all departures from a sphere have been multiplied by some huge factor. The Earth is much more spherical than this, but this just illustrates the departure from sphericity that the Earth has. All right. And so you see this great big dip in the Indian Ocean. You see this bulge over China. You can see a bit of a dip over northern Canada. And and it's not even constant in time. This thing, it varies on geological time scales, as you know, have mountain building. You have continental drift. That's millions of years, right. It also varies on shorter time scales because of glacial effects. So over the last 10,000 years or so, it's gradually changed because of the disappearance of the Laurentide Ice Sheet here. So Canada is gradually rebounding from the end of the last ice age 10,000 years ago, and it's still moving away from the center of the Earth because that weight has been lifted. Right. But for our purposes, for a daily tidal cycle, we can say this is the shape of the Earth. So what are the variations around that? Right. So here is a time series of sea level, right. Measured at some coastal station somewhere in England. And so what do you see when you look at that that time series. Well, the first thing you notice is that it varies quite quickly. So these are the days. This is about a month along the bottom. So within a day it's gone up and down twice basically. And so we have two highs and two lows every day. So we have a semi-diurnal tide. The second thing you notice is that the amplitude of this semi-diurnal tide varies throughout the month. Yeah. So you have some periods in the month where it's quite high amplitude and some periods where the amplitude is lower. And there are two of these maxima in the month. If you think back to when I was talking about interference between two waves, this is quite similar to that, right? We were talking about two different wave frequencies and the packet, the modulation packet. Remember that right. So if you have two frequencies which are very similar, they can combine to make something which is a much lower frequency which modulates the amplitude of that fast signal. This is a sign that we're looking at two different frequencies, tidal frequencies, which are both of them close to the semidiurnal frequency, but not exactly the same. So that's one way of looking at this. We'll come back to that later. There's a simpler explanation as well. But what could it be? I mean, what what could it be that's giving you two different frequencies. There's there's two things out there that are causing the tides, right? Two great big objects in the sky. One is up there. It's a beautiful day. You can see one of them now. Yeah. The the moon and the sun. Yeah. So there's two things they both contribute to the tides. Not exactly at the same frequency. So that's where you get this modulation. Right. But we'll come back to that.
Describe in detail the effect tidal nodes or amphidromic points have on the tide. Explain how they control tidal amplitude. What causes their fractal like appearance? Do they coincide with the highest high tides or anything else?
Tidal nodes or amphidromic points are points in the ocean where the tidal range is zero. These points are created by the interference of tidal waves as they move around the ocean basins. The tidal waves are created by the gravitational forces of the moon and the sun, and they move around the ocean basins in a circular pattern. As the waves move, they interfere with each other, creating areas where the tidal range is zero. The effect of tidal nodes or amphidromic points on the tide is that they control the tidal amplitude. The tidal amplitude is the difference between the high tide and the low tide. The closer a location is to a tidal node or amphidromic point, the smaller the tidal amplitude will be. Conversely, the further away a location is from a tidal node or amphidromic point, the larger the tidal amplitude will be. The fractal-like appearance of tidal nodes or amphidromic points is due to the complex interaction of tidal waves as they move around the ocean basins. The ocean basins are not uniform in shape, and the coastline and seafloor topography can create areas of interference that affect the movement of the tidal waves. This creates a complex pattern of nodes and antinodes that can appear fractal-like in nature. Tidal nodes or amphidromic points do not coincide with the highest high tides or any other specific tidal event. They are simply points in the ocean where the tidal range is zero. However, the location of tidal nodes or amphidromic points can have an impact on the tidal range at nearby locations. Areas that are close to a tidal node or amphidromic point will experience smaller tidal ranges, while areas that are further away will experience larger tidal ranges.
That sounds dry and boring so lets ask chat gpt to spice it up?
Imagine the ocean as a vast, swirling dance floor where the sun and moon lead an intricate ballet of waves. Tidal nodes, also known as amphidromic points, are unique spots on this dance floor where the tidal range - the difference between high and low tide - hits a mesmerizing pause: zero.
These special points emerge from the delicate interplay of tidal waves, orchestrated by the moon's and sun's gravitational pull. As these waves glide and twirl across the ocean's basins, they occasionally step on each other's toes, creating these zones of stillness.
The closer you are to a tidal node, the less dramatic the tides feel. It's like being near the eye of a storm, where things are calm and the tidal amplitude - the height difference between the high and low tides - is minimal. On the flip side, the further you drift from these points, the more the ocean's rhythm intensifies, leading to higher highs and lower lows of tides.
The patterns of these nodes and their counterpart antinodes, areas of maximum tidal range, resemble a fractal tapestry woven by nature. The irregular shapes of ocean basins, combined with the varying contours of coastlines and the ocean floor, contribute to this complex, ever-changing design.
It's fascinating to note that tidal nodes aren't tied to the extreme tidal events themselves; they don't mark the highest tides or any other specific tidal occurrence. They are simply the ocean's natural points of equilibrium where tidal movements neutralize each other. Yet, their presence subtly influences the tidal rhythms experienced along coastlines and in marine environments nearby.
In essence, tidal nodes or amphidromic points are the ocean's whispers, telling a story of celestial forces and underwater topography, a story that shapes the ebb and flow of our planet's lifeblood.
Question
Contents can you describe that math that foes into calculating the force in newtons to each part of earth that creates the tidal bulge?
Yes, the force in Newtons that creates the tidal bulge can be calculated using the formula: F = G _(m1_ m2 / r^2) where F is the force in Newtons, G is the gravitational constant (6.674 x 10^-11 N m^2/kg^2), m1 is the mass of one object (in this case, the moon), m2 is the mass of the other object (in this case, the Earth), and r is the distance between the centers of the two objects. To calculate the force that creates the tidal bulge, we need to consider the difference in gravitational force between the side of the Earth facing the moon and the opposite side of the Earth. This difference in force is given by: ΔF = G _(m1_ m2 / r1^2) - G _(m1_ m2 / r2^2) where r1 is the distance between the center of the Earth and the moon when it is directly overhead, and r2 is the distance between the center of the Earth and the moon when it is on the opposite side of the Earth. This difference in force creates a net gravitational force that pulls the water on the Earth's surface towards the moon, creating the tidal bulge. The actual calculation of the force in Newtons depends on the specific values of the masses and distances involved. For example, the mass of the moon is approximately 7.34 x 10^22 kg, and the average distance between the Earth and the moon is approximately 384,400 km. Plugging these values into the formula above, we can calculate the force in Newtons that creates the tidal bulge. However, it's important to note that this is a simplified calculation and that the actual forces involved in creating the tides are much more complex, involving the gravitational forces of both the moon and the sun, as well as the Earth's rotation and orbit.
Can you show me any and all math used in this specific pdf
Sure, here are some examples of the math used in the PDF file "Tides [Waves, Tidal Forces. lunar and Solar Tides, Tidal periods and Amplitudes, Tides in Ocean Basins].pdf":
- On page 7, the gravitational force between the Earth and the moon is given by: [ F_g = G \times \frac{m_1 \times m_2}{r^2} ] where: - ( F_g ) is the gravitational force - ( G ) is the gravitational constant (approximately (6.674 \times 10^{-11} , \text{N} , \text{m}^2/\text{kg}^2)) - ( m_1 ) is the mass of one object (e.g., the Earth) - ( m_2 ) is the mass of the other object (e.g., the moon) - ( r ) is the distance between the centers of the two objects
- On page 7, the centrifugal force is given by: [ F_c = \frac{m \times v^2}{r} ] where: - ( F_c ) is the centrifugal force - ( m ) is the mass of the object (e.g., the Earth) - ( v ) is the velocity of the object - ( r ) is the distance between the object and the center of rotation
- On page 7, the net force generating the tide is given by: [ F_{net} = F_g - F_c ] where: - ( F_g ) is the gravitational force between the Earth and the moon - ( F_c ) is the centrifugal force -
- On page 7, the formula for the gravitational force generating the tide is given by: [ F_t = G \times \frac{m_1 \times m_2 \times 2 \times R}{r^3} ] where: - ( F_t ) is the gravitational force generating the tide - ( G ) is the gravitational constant - ( m_1 ) is the mass of one object (e.g., the moon) - ( m_2 ) is the mass of the other object (e.g., the Earth) - ( R ) is the radius of the Earth - ( r ) is the distance between the centers of the two objects
- On page 10, Kepler's law of equal areas is used to calculate the speed of an object in an elliptical orbit: [ \frac{dA}{dt} = \frac{1}{2} r^2 \frac{d\theta}{dt} = \text{constant} ] where: - ( A ) is the area swept out by the object in a given time - ( r ) is the distance between the object and the focus of the ellipse - ( \theta ) is the angle between the object and the focus of the ellipse - ( t ) is time
Can you give a very detailed explanation of how the interfering gravitation forces compete to destructively interfere and constructively interfere with each other, and explained what that actually means.
Sure, I'd be happy to explain how the interfering gravitational forces of the moon and the sun create constructive and destructive interference, and what that means for tides. The gravitational forces of the moon and the sun both act on the Earth's oceans, creating tidal bulges. The gravitational force of the moon is stronger than that of the sun, so the lunar tidal bulge is larger than the solar tidal bulge. However, the positions of the moon and the sun relative to the Earth are constantly changing, which means that the tidal bulges they create are also changing. When the gravitational forces of the moon and the sun are aligned, they create a combined tidal bulge that is larger than either the lunar or solar tidal bulge alone. This is known as constructive interference, and it results in higher high tides and lower low tides. These are called spring tides, and they occur twice a month, during the full and new moons. On the other hand, when the gravitational forces of the moon and the sun are working against each other, they create a smaller combined tidal bulge. This is known as destructive interference, and it results in lower high tides and higher low tides. These are called neap tides, and they occur twice a month, during the first and third quarter moons. The interference between the gravitational forces of the moon and the sun is due to the changing positions of the two bodies relative to the Earth. As the moon orbits the Earth, it carries its tidal bulge with it, causing it to move around the Earth once every 24 hours and 50 minutes. This is known as a lunar day. The sun also has a tidal effect on the Earth, but because it is so much further away than the moon, its effect is much weaker. However, the sun's position relative to the Earth also changes over time, due to the Earth's orbit around the sun. This means that the positions of the lunar and solar tidal bulges are constantly changing relative to each other, leading to the constructive and destructive interference that creates spring and neap tides. In summary, the interfering gravitational forces of the moon and the sun create constructive and destructive interference, which results in the spring and neap tides that we observe on Earth. This interference is due to the changing positions of the two bodies relative to the Earth, and it is a complex phenomenon that is influenced by many factors, including the Earth's rotation and orbit.
Tide Dynamics Dynamic Theory of Tides.
Kelly, K. (2005). "Tide Dynamics Dynamic Theory of Tides."
The section discusses the [equilibrium theory](https://en.wikipedia.org/?curid=45938) of [tides](https://en.wikipedia.org/?curid=30718) and the underlying physics involved in their generation. It explains that the shape of the [sea surface](https://en.wikipedia.org/?curid=899880) is assumed to be in equilibrium with the forcing, which leads to [lateral movement](https://en.wikipedia.org/?curid=8997567) of sea level bulges through the ocean as a surface gravity wave. Laplace rearranged the rotating shallow water equations to create the Laplace tidal equations, which describe the horizontal forces governing [tides](https://en.wikipedia.org/?curid=30718). The tide producing forces are a small fraction of the total gravity magnitude, maintaining a hydrostatic vertical balance. Tides are forced by a combination of pressure gradient force and [tractive force](https://en.wikipedia.org/?curid=3113840) and propagate like [](https://en.wikipedia.org/?curid=935830)[Kelvin waves](https://en.wikipedia.org/?curid=935830). The section also mentions the ability to predict [tides](https://en.wikipedia.org/?curid=30718) using numerical ocean tidal models that assimilate observational data, providing results in the form of maps of phase and amplitude for each tidal component. These components are then added together to form the total tidal sea level variation. [ 1 ]
**Tidal parameters**
The study discusses different [tidal parameters](https://en.wikipedia.org/?curid=30718) associated with tidal constituents. These parameters include co-range lines, which connect locations with the same tidal range, and [co-tidal lines](https://en.wikipedia.org/?curid=30718), which connect points with the same phase, indicated in hours of lag of high tide after the moon's transit over the Greenwich meridian or as a phase relative to Greenwich. Amphidromic points are also defined as points where the tidal range is zero, and wave crests move around them once per [tidal cycle](https://en.wikipedia.org/?curid=30718). The study emphasizes the cyclonic progression around amphidromes near the coast, particularly in the Northern Hemisphere. The paper illustrates co-range and [co-tidal lines](https://en.wikipedia.org/?curid=30718) for the M2 tide, highlighting the presence of amphidromes in the North Atlantic and the Pacific, as well as the southward propagation along the west coast of South America and the northward propagation along the west coast of North America and Africa. The findings suggest the significance of these [tidal parameters](https://en.wikipedia.org/?curid=30718) in understanding the complex tidal patterns, particularly in regions with distinct amphidromes and coastal propagation. [ 2 ]
**Tides in [bays](https://en.wikipedia.org/?curid=3935892) and [channels](https://en.wikipedia.org/?curid=40860)**
The research paper discusses the behavior of [tides](https://en.wikipedia.org/?curid=30718) in [bays](https://en.wikipedia.org/?curid=3935892) and [channels](https://en.wikipedia.org/?curid=40860). It highlights that [tides](https://en.wikipedia.org/?curid=30718) in the open ocean and along boundaries take the form of progressive Poincaré or [](https://en.wikipedia.org/?curid=935830)[Kelvin waves](https://en.wikipedia.org/?curid=935830). In the English Channel, tidal propagation from the North Atlantic eastward is observed, with higher tidal ranges on the right-hand side due to the Kelvin wave character. The response of a bay or inlet depends on its width compared with the wavelength of the tidal response. Wide [bays](https://en.wikipedia.org/?curid=3935892) allow tidal propagation around boundaries, with the requirement that the bay must be wide enough relative to its depth to prevent interference between incoming and outgoing waves. Narrow [bays](https://en.wikipedia.org/?curid=3935892) result in reflected waves and [standing waves](https://en.wikipedia.org/?curid=41741), with the possibility of interference and the establishment of natural frequencies. The paper also addresses the structure of [tidal currents](https://en.wikipedia.org/?curid=30718), including maximum current speeds and the potential for [standing waves](https://en.wikipedia.org/?curid=41741) when the propagating tide meets a reflection. It discusses the characteristics of [standing waves](https://en.wikipedia.org/?curid=41741), including the presence of [anti](https://en.wikipedia.org/?curid=998070)[nodes](https://en.wikipedia.org/?curid=21707) as locations where the standing wave has maximum amplitude. The section provides insights into the dynamics of [tides](https://en.wikipedia.org/?curid=30718) in [bays](https://en.wikipedia.org/?curid=3935892) and [channels](https://en.wikipedia.org/?curid=40860), considering factors such as width, depth, and reflection. [ 3 ]
**2:**
The section discusses the behavior of [standing waves](https://en.wikipedia.org/?curid=41741) and [tidal currents](https://en.wikipedia.org/?curid=30718) in different environments, highlighting the significance of [nodes](https://en.wikipedia.org/?curid=21707) and [anti](https://en.wikipedia.org/?curid=998070)[nodes](https://en.wikipedia.org/?curid=21707) in determining wave amplitudes and velocities. Nodes, where there is zero amplitude due to destructive interference, are found in closed harbors and at the center of a basin, while [anti](https://en.wikipedia.org/?curid=998070)[nodes](https://en.wikipedia.org/?curid=21707) occur at solid walls. The presence of [nodes](https://en.wikipedia.org/?curid=21707) and [anti](https://en.wikipedia.org/?curid=998070)[nodes](https://en.wikipedia.org/?curid=21707) is determined by the basin's geometry. In the context of [tidal currents](https://en.wikipedia.org/?curid=30718), maximum vertical velocities are observed at the [anti](https://en.wikipedia.org/?curid=998070)[nodes](https://en.wikipedia.org/?curid=21707), while horizontal velocities are highest at the [nodes](https://en.wikipedia.org/?curid=21707), such as the inlet opening in a channel. The section also explains that maximum [tidal currents](https://en.wikipedia.org/?curid=30718) occur between the extremes of sea level, as water fills or empties the [tidal prism](https://en.wikipedia.org/?curid=24878341). Furthermore, it defines the average [tidal prism](https://en.wikipedia.org/?curid=24878341) as the volume difference between mean high water and mean low water and explains the concept of [flood tide](https://en.wikipedia.org/?curid=30718) (rising sea level) and [ebb tide](https://en.wikipedia.org/?curid=30718) (falling sea level). Overall, the section provides insights into the behavior of [standing waves](https://en.wikipedia.org/?curid=41741) and [tidal currents](https://en.wikipedia.org/?curid=30718) in different settings, emphasizing the role of [nodes](https://en.wikipedia.org/?curid=21707), [anti](https://en.wikipedia.org/?curid=998070)[nodes](https://en.wikipedia.org/?curid=21707), and basin geometry in influencing wave amplitudes and velocities, as well as the temporal variation of [tidal currents](https://en.wikipedia.org/?curid=30718). [ 5 ]
**Resonance.**
The research paper examines the [resonance](https://en.wikipedia.org/?curid=41660) phenomenon in a closed harbor and an open harbor. In the closed harbor, the simplest standing wave structure with [anti](https://en.wikipedia.org/?curid=998070)[nodes](https://en.wikipedia.org/?curid=21707) at each wall is one-half wavelength long, represented by L = λ/2 where L is the distance between the walls. In the open harbor, the simplest wave structure is one-quarter wavelength long, represented by L = λ/4 where L is the length of the harbor. By combining these spatial scales with the phase speed of the shallow water wave, the period for this wave can be determined using T = 2L/gH, where T is the period, g is the acceleration due to gravity, and H is the water depth. The natural periods for n [nodes](https://en.wikipedia.org/?curid=21707) can also be determined, and it is found that the natural period of oscillation is very close to the period of the M2 tide. This suggests that the harbor will be very near [resonance](https://en.wikipedia.org/?curid=41660), resulting in a high tide at the head of the bay. Overall, the paper provides insights into the [resonance](https://en.wikipedia.org/?curid=41660) behavior of waves in closed and open harbors based on their geometry, spatial scales, and natural periods, and discusses the implications of [resonance](https://en.wikipedia.org/?curid=41660) on tide behavior. [ 6 ]
**Mixed geometries**
The mixed geometries section of the research paper explores the behavior of [tides](https://en.wikipedia.org/?curid=30718) in [bays](https://en.wikipedia.org/?curid=3935892) and inlets that exhibit a combination of open and closed harbor characteristics. It delves into the impact of [tides](https://en.wikipedia.org/?curid=30718) propagating into wide [bays](https://en.wikipedia.org/?curid=3935892), where the Kelvin wave effect influences the coastline and the exponential offshore decay shows rotational effects. The deformation radius relative to the width of the bay is calculated to determine the full effects of rotation. Additionally, the paper discusses the behavior of [tides](https://en.wikipedia.org/?curid=30718) in very narrow [bays](https://en.wikipedia.org/?curid=3935892), where the M 2 tide sets up a resonant standing wave, leading to the formation of co-tidal and co-range lines and highlighting the distribution of high and low [tides](https://en.wikipedia.org/?curid=30718). The intermediate inlet width is also explored, with the characteristics of the tide being a combination of the Kelvin wave case and the standing wave case. The paper goes on to explain that the boundaries of the inlet will strongly resemble the Kelvin wave case, while the center axis will resemble the standing wave case, creating an out-of-phase relationship with high and low amplitude [tides](https://en.wikipedia.org/?curid=30718). Furthermore, it discusses how the nodal line in cases where rotation is not important will become a nodal point in the center of the channel around which the tide progresses, termed an amphidrome
"Tidal measurements, analysis and modeling."
**Tide Prediction Methods**
The research paper discusses different methods for predicting tides. The first method is [astronomical prediction](https://en.wikipedia.org/?curid=37001823), also known as [equilibrium tide](https://en.wikipedia.org/?curid=30718). However, this method has [limitations](https://en.wikipedia.org/?curid=9401640) as it neglects factors such as topography and the coast. The second method is statistical methods, particularly [harmonic analysis](https://en.wikipedia.org/?curid=14147). However, this method also has [limitations](https://en.wikipedia.org/?curid=9401640) as it requires observations and cannot predict [tidal currents](https://en.wikipedia.org/?curid=30718). The third method is hydrodynamic models, which have both advantages and [limitations](https://en.wikipedia.org/?curid=9401640). The [limitations](https://en.wikipedia.org/?curid=9401640) include the computational demand of high-resolution models and the need for boundary conditions. However, these models can predict water level and [tidal currents](https://en.wikipedia.org/?curid=30718), and can also incorporate factors such as wind, rivers, storm surge, and waves. The fourth method discussed is related to coastal ocean observations, including data from tide gauges, [harmonic analysis](https://en.wikipedia.org/?curid=14147) from [historical data](https://en.wikipedia.org/?curid=7990) (some dating back approximately 100 years), and regional computer models forced by open boundary conditions. These methods are used to predict tides and offer a comprehensive understanding of the [dynamics](https://en.wikipedia.org/?curid=8386) of coastal ocean systems. However, it is important to consider the advantages and [limitations](https://en.wikipedia.org/?curid=9401640) of each method when choosing the most appropriate approach for predicting tides. [ 8 ]
**Tide Prediction Methods**
The research paper evaluates various tide prediction methods and their [limitations](https://en.wikipedia.org/?curid=9401640) and advantages. The first method discussed is [astronomical prediction](https://en.wikipedia.org/?curid=37001823), also known as [equilibrium tide](https://en.wikipedia.org/?curid=30718), which has [limitations](https://en.wikipedia.org/?curid=9401640) in neglecting topography and coast, among other factors. The second method is statistical methods, specifically [harmonic analysis](https://en.wikipedia.org/?curid=14147), which requires observations and cannot predict [tidal currents](https://en.wikipedia.org/?curid=30718). The third method is hydrodynamic models, which despite being computationally demanding and requiring boundary conditions, have the advantage of being able to predict water level and [tidal currents](https://en.wikipedia.org/?curid=30718) and include various factors such as wind, rivers, storm surge, and waves. The paper also highlights the use of coastal ocean observations from tide gauges, [harmonic analysis](https://en.wikipedia.org/?curid=14147) from [historical data](https://en.wikipedia.org/?curid=7990) (some spanning approximately 100 years), and regional computer models forced by open boundary conditions as valid tide prediction methods. The discussion emphasizes the [limitations](https://en.wikipedia.org/?curid=9401640) and advantages of each method, providing a comprehensive overview of the strengths and weaknesses of each approach. Overall, the paper sheds light on the various tide prediction methods and their associated considerations, providing valuable insights for further research and practical applications in the field. [ 8 ]
**Overview of Predicting Tides**
The scientific article provides an overview of methods and considerations for predicting [tides](https://en.wikipedia.org/?curid=30718). It discusses historical challenges in tidal measurements and emphasizes the importance of long-term records for calculating sea level rise trends. The article outlines various methods for predicting [tides](https://en.wikipedia.org/?curid=30718), including [astronomical prediction](https://en.wikipedia.org/?curid=37001823), statistical methods, and hydrodynamic models. It distinguishes between predicting [tides](https://en.wikipedia.org/?curid=30718) in coastal ocean versus open ocean, highlighting the reliance on long-term tide gauge data and [harmonic analysis](https://en.wikipedia.org/?curid=14147) for coastal predictions. The article discusses tidal [harmonic analysis](https://en.wikipedia.org/?curid=14147) and the development of tidal constituents, with a focus on separating water level signals into tidal and sub-tidal components. [ 8 ]
**Dynamic Tidal Prediction and Numerical Modeling**
It also delves into dynamic tidal prediction, emphasizing the need to consider detailed [bottom topography](https://en.wikipedia.org/?curid=164974), [bottom friction](https://en.wikipedia.org/?curid=1714223), [Coriolis effect](https://en.wikipedia.org/?curid=7783), and [wind stress](https://en.wikipedia.org/?curid=15452231) in coastal ocean and estuarine [tides](https://en.wikipedia.org/?curid=30718). The article describes the use of vertically averaged equations and numerical models for predicting [tides](https://en.wikipedia.org/?curid=30718), using examples such as Massachusetts Bay and Cook Inlet. Additionally, it highlights the importance of basin-scale models in providing boundary conditions for regional high-resolution models. [ 19 ]
Furthermore, the article explores examples of tidal simulations in specific geographical locations, such as Cook Inlet, Alaska, emphasizing the impact of geographical features on tidal patterns. It discusses the amplification of [tides](https://en.wikipedia.org/?curid=30718) and the resonance effect in specific inlets. The article also delves into the use of [remote sensing](https://en.wikipedia.org/?curid=228148) and 3D topography in mapping [tidal flats](https://en.wikipedia.org/?curid=551217), as well as modeling [tidal inundation](https://en.wikipedia.org/?curid=24878341) and its impact on water level. [ 31325152535455 ]
**Tides' Influence on Mixing and Temperature**
The impact of [tides](https://en.wikipedia.org/?curid=30718) on [mixing](https://en.wikipedia.org/?curid=255068) and [temperature](https://en.wikipedia.org/?curid=20647050) is also discussed, along with the potential link between [tides](https://en.wikipedia.org/?curid=30718) and beluga whale movements. The article concludes by teasing topics for the next class, including modeling global [tides](https://en.wikipedia.org/?curid=30718) and tools for studying and modeling [tides](https://en.wikipedia.org/?curid=30718), and it includes a reminder about an upcoming homework assignment. [ 58 ]
**Conclusion and Overview**
Overall, the article provides a comprehensive overview of predicting [tides](https://en.wikipedia.org/?curid=30718), encompassing historical challenges, various prediction methods, dynamic tidal prediction, numerical modeling, geographical impacts, and related phenomena such as [tidal inundation](https://en.wikipedia.org/?curid=24878341) and its influence on [temperature](https://en.wikipedia.org/?curid=20647050) and [mixing](https://en.wikipedia.org/?curid=255068). [ 8 ]
"Tidal Constituents Of Modern and Ancient Tidal Rhythmic Criteria."
The paper discusses the [relationship](https://en.wikipedia.org/?curid=26028) between [laminae](https://en.wikipedia.org/?curid=5240655), tidal current strength, and [tidal range](https://en.wikipedia.org/?curid=3895284), and how changes in tidal current strengths are reflected in the thicknesses of [vertically stacked](https://en.wikipedia.org/?curid=3555585) [laminae](https://en.wikipedia.org/?curid=5240655). It highlights the presence of modern and ancient tidal rhythmites on every continent except Antarctica and their significance in reconstructing ancient paleogeographies and estimating paleo[tidal range](https://en.wikipedia.org/?curid=3895284)s. The paper emphasizes the necessity to understand tidal cycles for interpreting rock records and explains the importance of both [equilibrium](https://en.wikipedia.org/?curid=37425) and dynamic tidal models for studying tides and tidal deposits. Additionally, it addresses the complexity of neap-spring or fortnightly tidal cycles and the misconception of these cycles in college-level textbooks. The paper also touches on the generation of tides by the gravitational forces of the Moon and the Sun, and the impact of [diurnal](https://en.wikipedia.org/?curid=3104473), semi[diurnal](https://en.wikipedia.org/?curid=3104473), and [semiannual](https://en.wikipedia.org/?curid=477069) tidal cycles on sediment deposition. It explains the different tidal periods and their correlation with astronomical effects, and the influence of the Moon's declination and its changing distance from the Earth on tidal behavior. The presence of tropical neap-spring tides, not predicted by [equilibrium](https://en.wikipedia.org/?curid=37425) tidal theory, is also highlighted. The paper aims to provide a thorough understanding of ancient tidal systems and emphasizes the necessity of understanding both [equilibrium](https://en.wikipedia.org/?curid=37425) and dynamic tidal models for studying tides and tidal deposits. [ 2 ]
**Seania nual (1X2.6 Days)**
The section discusses the complexities of tidal cycles, focusing on the differences between [synodic](https://en.wikipedia.org/?curid=241485), tropical, and [anomalistic](https://en.wikipedia.org/?curid=7056993) periods. The [equilibrium](https://en.wikipedia.org/?curid=37425) tidal model explains the driving forces that cause tides but does not fully explain real-world tides, as there are discrepancies such as [diurnal](https://en.wikipedia.org/?curid=3104473) tides in unexpected regions and neap-spring tidal cycles. More than 100 tidal constituents have been identified, with seven accounting for over 80% of any real-world tide. The paper explains the concept of tidal constituents and their influence on resulting tides in specific areas within the Earth's oceans. It also provides examples of how changes in the relative intensity of tidal constituents along a coast affect resulting tides. Furthermore, it discusses the significance of the dynamic tidal model in better understanding and explaining real-world tides, allowing for specific comparisons to be made to real-world analogues. The research identifies the dominance of certain tidal constituents in different tidal rhythmite packages, shedding light on the changes in tidal dynamics within specific geological periods. Overall, the paper emphasizes the importance of incorporating the dynamic tidal model to better comprehend the complexities of tidal cycles and their geological implications. [ 78 ]
The tidal periods and their correlation with astronomical effect
The article discusses six tidal periodicities, as indicated in Figures 1.1-1.6, which are associated with astronomical influences. These include
diurnal inequality,
the half-synodic month,
the tropical month,
the anomalistic month,
the semiannual convergence,
and the nodal cycle.
Each of these periodicities is influenced by different astronomical factors, such as the distance and declination of the Moon and its orbit relative to the Earth, as well as the interactions between the Earth, Moon, and Sun. The paper also discusses how each of these tidal periods can be recognized and analyzed in both modern and ancient tidal rhythmites, offering insights into paleogeographies, paleoclimates, and tidal range estimation.
The traditional equilibrium tidal theory and the dynamic tidal theory are two models used to understand and explain the behavior of tides. Here's a detailed comparison of the two:
Traditional Equilibrium Tidal Theory:
1. Assumptions: This theory is based on the assumption that the ocean responds instantaneously to the gravitational forces of the moon and the sun. It assumes that the ocean reaches equilibrium with the tidal forces, resulting in a predictable and stable tidal pattern.
2. Simplified Model: The traditional equilibrium tidal theory simplifies the complex interactions between the Earth, moon, and sun into a static model. It considers the Earth as a rigid body with a uniform ocean depth, neglecting factors such as the Earth's rotation, ocean basin shapes, and frictional effects.
3. Predictability: This model provides a straightforward way to predict tidal behavior based on the positions of the moon and the sun. It allows for the calculation of tidal heights and times using harmonic analysis, which involves decomposing the tidal signal into a sum of sinusoidal components with specific frequencies and amplitudes.
4. Limitations: While the traditional equilibrium tidal theory is useful for making tidal predictions over long time scales, it does not account for certain dynamic phenomena such as tidal currents, the effects of coastal geography, and the influence of atmospheric pressure on tides.
Dynamic Tidal Theory:
7. Consideration of Dynamic Factors: The dynamic tidal theory takes into account the dynamic nature of tidal phenomena, considering factors such as the Earth's rotation, the shape of ocean basins, frictional effects, and the influence of atmospheric and oceanic circulation patterns.
8. Time-Dependent Model: Unlike the equilibrium tidal theory, the dynamic tidal theory recognizes that the ocean's response to tidal forces is time-dependent and can exhibit complex variations over different time scales.
9. Incorporation of Nonlinear Effects: This model incorporates nonlinear effects, such as resonance phenomena in coastal areas and the amplification of tides in certain ocean basins, which are not accounted for in the traditional equilibrium tidal theory.
10. Applications: The dynamic tidal theory is particularly valuable for understanding short-term variations in tides, predicting tidal currents, and studying the impact of tides on coastal and estuarine environments.
In summary, the traditional equilibrium tidal theory provides a simplified and predictable model for understanding long-term tidal behavior, while the dynamic tidal theory offers a more comprehensive and nuanced approach that considers the dynamic and nonlinear aspects of tidal phenomena, making it particularly useful for studying short-term variations and complex tidal interactions in specific geographical settings. Or, In other words, equilibrium tides are kinematic descriptions of the forces involved in the celestial procession that pay no attention to the actual factors controlling tides on earth, and dynamical tides detail how the water is actually pushed around on earth without incorporating the mathamagical descriptions of the gravitational forces supposedly causing all of this.
Chat GBT Paper
**Introduction** Tidal phenomena have long fascinated scientists, leading to the development of various theories to explain this complex oceanic behavior. Among these, the Traditional Equilibrium Theory and the Dynamic Tide Theory stand out as two pivotal frameworks. This paper aims to compare these theories, highlighting their principles, strengths, and limitations in explaining the mechanics of tides.
**Traditional Equilibrium Tide Theory**
_Overview_: The Traditional Equilibrium Tide Theory, rooted in Newtonian mechanics, proposes that the gravitational pull of the moon and the sun generates tides on Earth. This theory conceptualizes the Earth and its water as part of a two-body system, where tidal forces create bulges of water both towards and away from the celestial bodies.
_Strengths_: This theory simplifies the understanding of tidal forces and their celestial origins. It effectively explains the occurrence of spring and neap tides based on the relative positions of the Earth, moon, and sun.
_Limitations_: Its primary limitation lies in its oversimplified model that doesn't account for Earth's rotation, ocean depth variations, and the continents' interference, which in reality, significantly affect tidal patterns.
**Dynamic Tide Theory**
_Overview_: The Dynamic Tide Theory, a more contemporary approach, considers the ocean's response to tidal forces as a complex, dynamic system. This theory incorporates the effects of Earth's rotation, the ocean's bathymetry, and the interactions of tidal waves with continental coastlines and ocean basins.
_Strengths_: It provides a more comprehensive understanding of the variations in tidal patterns across different geographic locations. The theory explains complex phenomena such as amphidromic points, tidal currents, and the formation of tidal waves.
_Limitations_: The complexity of this theory poses challenges in terms of computational requirements for predicting tides and requires extensive empirical data for accurate modeling.
**Comparative Analysis**
1. **Conceptual Approach**: While the Traditional Equilibrium Theory offers a foundational understanding based on gravitational forces, the Dynamic Tide Theory provides a more nuanced approach, considering the fluid dynamics of the oceans.
2. **Accuracy in Prediction**: Dynamic Tide Theory is generally more accurate in predicting local tidal events due to its consideration of various influencing factors, unlike the Traditional Equilibrium Theory, which is more generic and less precise.
3. **Applicability**: The equilibrium model is more suited for educational purposes and simple predictions, while the dynamic model is utilized in professional and navigational settings where detailed information is crucial.
4. **Historical Development**: The evolution from the Traditional Equilibrium to the Dynamic Tide Theory signifies a shift from a simplistic to a more holistic understanding of tides, reflecting advancements in computational and oceanographic sciences.
**Conclusion**
In conclusion, both the Traditional Equilibrium and Dynamic Tide Theories have significantly contributed to our understanding of tides. The Traditional Equilibrium Theory lays the groundwork for understanding the celestial influences on tides, while the Dynamic Tide Theory advances this understanding by incorporating the complexities of ocean dynamics. As our computational capabilities and understanding of oceanography evolve, the Dynamic Tide Theory is likely to continue to provide more accurate and detailed insights into tidal behaviors.
# Key Points
- The article compares the traditional equilibrium-tidal theory model with the dynamic tidal theory, explaining how tides are influenced by the [gravitational forces](https://en.wikipedia.org/?curid=2170421) of the Moon and Sun in the former, and by a series of phantom "satellites" creating tidal waves that move around an [amphidromic point](https://en.wikipedia.org/?curid=336138) in the latter.
- What are phantom "satellites" creating tidal waves
- The concept of "phantom satellites" creating tidal waves is likely a reference to the hypothetical scenario where celestial bodies other than the moon and the sun, often referred to as "phantom satellites," could potentially influence tidal forces. In the context of tidal theory, these phantom satellites are theoretical bodies that, if they existed, would exert gravitational forces on the Earth and contribute to the generation of tidal waves. In traditional tidal theory, the gravitational forces of the moon and the sun are the primary drivers of tidal phenomena. However, some discussions in theoretical physics and astronomy explore the potential effects of additional celestial bodies, sometimes referred to as phantom satellites, on tidal forces. These hypothetical bodies are not actual physical satellites but are used in theoretical models to explore the potential influence of additional gravitational forces on tidal behavior. It's important to note that the concept of phantom satellites in the context of tidal waves is largely theoretical and speculative. In practical tidal predictions and analyses, the gravitational forces of the moon and the sun are the dominant factors considered. The inclusion of phantom satellites is more of a theoretical exercise to explore the potential impacts of additional gravitational influences on tidal behavior, rather than a reflection of observed celestial bodies.
- It highlights the use of [tidal rhythmites](https://en.wikipedia.org/?curid=10564558), [sedimentary structures](https://en.wikipedia.org/?curid=16398712), to reconstruct ancient paleogeographies, estimate paleotidal ranges, and understand tidal systems. It emphasizes the need to understand both equilibrium and dynamic tidal models for interpreting the rock record accurately. [ 11516.1172 ]
- The article advocates for the practical implications of the dynamic tidal model, such as estimating changes in Earth-Moon distance, studying tidal basin dynamics, and understanding the impact of tides on [biological systems](https://en.wikipedia.org/?curid=5780599), and presents examples of extracting tidal constituents from the rock record to provide insights into changes in tidal dynamics and the Earth-Moon system. [ 1158 ]
# Synopsis
**Oceanic Tides and Tidal Theories**
The scientific article discusses the origin of oceanic tides, challenging the traditional equilibrium-tidal theory model with the dynamic tidal theory. The equilibrium model explains tides based on changes in two tidal bulges created by [gravitational forces](https://en.wikipedia.org/?curid=2170421) of the Moon and Sun, while the dynamic model models tides as a result of the combined effects of a series of phantom "satellites" generating their own tidal wave. These tidal waves move around an [amphidromic point](https://en.wikipedia.org/?curid=336138), and the tide-raising potential for any locality on Earth is conceptualized as the summation of amplitudes of specific tidal constituents determined by the geometries of ocean basins. The article demonstrates how [tidal rhythmites](https://en.wikipedia.org/?curid=10564558), [sedimentary structures](https://en.wikipedia.org/?curid=16398712) recording cyclic variations associated with lunar/solar cycles, can be used to reconstruct ancient paleogeographies, estimate paleotidal ranges, and understand tidal systems. It emphasizes the need to understand both equilibrium and dynamic tidal models for interpreting the rock record accurately. The dynamic tidal model has practical implications for estimating changes in Earth-Moon distance, studying tidal basin dynamics, and understanding the impact of tides on [biological systems](https://en.wikipedia.org/?curid=5780599). The article also presents examples of extracting tidal constituents from the rock record and argues that a basic understanding of the dynamic tidal system creates [research opportunities](https://en.wikipedia.org/?curid=400598) not accessible through the equilibrium tidal theory alone. [ 1 ]
**Advantages of Dynamic Tidal Theory**
Overall, the article advocates for a shift from the traditional equilibrium tidal theory model to the dynamic tidal theory for a more comprehensive understanding of tidal systems and their ancient records, emphasizing the practical utility and significance of the dynamic model in various scientific fields. The examples provided demonstrate how the understanding of tidal constituents extracted from the rock record can provide insights into changes in tidal dynamics and the Earth-Moon system, contributing to a deeper understanding of Earth's [geological history](https://en.wikipedia.org/?curid=186752). [ 1158 ]
## Introduction to ocean tides
# Key Points
- The paper discusses the renewed interest in understanding [tidal energy](https://en.wikipedia.org/?curid=325060) [dissipation](https://en.wikipedia.org/?curid=164572) and its potential contributions to oceanic mixing and [thermohaline circulation](https://en.wikipedia.org/?curid=387457). [ 1 ]
- It provides a comprehensive overview of tidal generating mechanisms, mathematical expressions for tide generating potential, and the intricate calculations and modulations involved in analyzing tidal frequencies and [components](https://en.wikipedia.org/?curid=40934). [ 1 ]
- The paper presents a [spectrum](https://en.wikipedia.org/?curid=29329) for equilibrium tides, showcasing the frequencies of tidal [components](https://en.wikipedia.org/?curid=40934) and their fine splitting related to cycles per month and [year](https://en.wikipedia.org/?curid=34341), offering a detailed insight into the complex nature of [tidal energy](https://en.wikipedia.org/?curid=325060) and its [dissipation](https://en.wikipedia.org/?curid=164572) in the ocean. [ 1 ]
# Synopsis
The paper discusses the renewed interest in understanding the [dissipation](https://en.wikipedia.org/?curid=164572) of [tidal energy](https://en.wikipedia.org/?curid=325060) in the ocean, as well as the possibility of [tidal energy](https://en.wikipedia.org/?curid=325060) exciting far-traveling internal waves and contributing to oceanic mixing and [thermohaline circulation](https://en.wikipedia.org/?curid=387457). The introductory lecture reviews tidal generating mechanisms and derives a mathematical expression for the tide generating potential. It explains that tidal oscillations are the response of the ocean and the Earth to the gravitational pull of celestial bodies other than the Earth, and their temporal response is very linear, allowing for localized tidal prediction. The paper explores tidal records from Port Adelaide, Australia, highlighting characteristics of tidal oscillations related to astronomical forcings. [ 1 ]
**Tidal Generating Potential and Celestial Influence**
The paper further delves into the tidal generating potential, emphasizing the influence of celestial bodies such as the Moon and the Sun, and how their gravitational force leads to the formation of tides. It clarifies common misconceptions about the causes of tides and elaborates on the impact of Earth's movement relative to these bodies. The paper also discusses the obstruction of tidal wave propagation by continents and bottom topography, and the use of satellite altimetry to obtain precise maps for different astronomical [components](https://en.wikipedia.org/?curid=40934). [ 13 ]
**Calculation and Modulation of Tidal Force**
Moreover, the paper details the calculation of tidal force exerted by the Moon or Sun on the Earth, expressing the tidal potential in geographical coordinates. It delves into the effects of variations in the declination of the tide generating body and the orbital distance of the Moon, highlighting the complex nature of calculating and understanding tidal potentials. The analysis involves Fourier decomposition and modulation of basic tidal frequencies due to variations in declination and distances to the tide generating bodies. [ 173569 ]
**Spectrum for Equilibrium Tides**
Additionally, the paper presents a [spectrum](https://en.wikipedia.org/?curid=29329) for equilibrium tides, showcasing the frequencies of tidal [components](https://en.wikipedia.org/?curid=40934) and their fine splitting related to cycles per month and [year](https://en.wikipedia.org/?curid=34341). This [spectrum](https://en.wikipedia.org/?curid=29329) provides a comprehensive visualization of the various frequencies associated with equilibrium tides, offering a detailed insight into the complex nature of [tidal energy](https://en.wikipedia.org/?curid=325060) and its [dissipation](https://en.wikipedia.org/?curid=164572) in the ocean. [ 1 ]
In summary, the paper thoroughly examines the phenomenon of oceanic tides, emphasizing the renewed interest in understanding [tidal energy](https://en.wikipedia.org/?curid=325060) [dissipation](https://en.wikipedia.org/?curid=164572) and its potential contributions to oceanic mixing and [thermohaline circulation](https://en.wikipedia.org/?curid=387457). It provides a comprehensive overview of tidal generating mechanisms, mathematical expressions for tide generating potential, and the intricate calculations and modulations involved in analyzing tidal frequencies and [components](https://en.wikipedia.org/?curid=40934). [ 1 ]
**Introduction**
The introduction discusses the renewed interest in the scientific community in studying oceanic tides, particularly focusing on the [dissipation](https://en.wikipedia.org/?curid=164572) of [tidal energy](https://en.wikipedia.org/?curid=325060) in the ocean. While it was previously believed that tidal phenomena were well understood, recent research has prompted a reevaluation of this assumption. The paper aims to review the tidal generating mechanisms and establish a mathematical expression for the tide generating potential. Research in the seventies indicated that [tidal energy](https://en.wikipedia.org/?curid=325060) could excite [internal waves](https://en.wikipedia.org/?curid=900160) in the ocean, which in turn could contribute to oceanic mixing and potentially drive the [thermohaline circulation](https://en.wikipedia.org/?curid=387457). The renewed interest in studying [tidal energy](https://en.wikipedia.org/?curid=325060) [dissipation](https://en.wikipedia.org/?curid=164572) and its potential impact on oceanic processes highlights the importance of further scientific investigation in this area. [ 1 ]
**Tide Generating Forces**
The [tidal oscillations](https://en.wikipedia.org/?curid=78471) in the ocean are a response to the gravitational pull of celestial bodies, causing changes in gravitational force over time and space on Earth's surface. Tidal prediction is facilitated by the linear temporal response of the ocean, allowing records to be interpreted as a combination of periodic components with frequencies associated with celestial body movements. Spatial response is influenced by [continents](https://en.wikipedia.org/?curid=19630739) and [bottom topography](https://en.wikipedia.org/?curid=164974). The tidal record from Port Adelaide, Australia, exhibits characteristics like semi-diurnal components, spring-neap cycles associated with the Moon's phases, and daily inequality linked to the Moon's position. The paper aims to explain the principles of tidal forcing, the origin of forcing terms, and the derivation of tide generating potential. [ 1 ]
**Tidal Forcing**
The section discusses the tidal forcing by the Moon on Earth, highlighting its influence on the formation of tides. It explains the gravitational effects of the Moon, Earth's rigid body, and the resultant bulging of the ocean on opposite hemispheres. The role of centrifugal force due to the Earth's spin in creating semi-diurnal tides is detailed. The paper goes on to discuss the impact of the elliptical orbit of the Moon, the interplay between lunar and solar tidal components, and the daily inequality due to the inclination of the lunar orbital plane to the equatorial plane. Additionally, it addresses the usefulness of decomposing tidal forces into harmonics for precise tidal predictions. The section emphasizes the linear response of the oceans to tidal forces, providing insights into tidal variations and the importance of astronomical considerations for tidal predictions. [ 3 ]
**Spatial Structure of the Tides**
The spatial structure of tides is influenced by the [Earth's rotation](https://en.wikipedia.org/?curid=4396171), [continents](https://en.wikipedia.org/?curid=19630739), and [bottom topography](https://en.wikipedia.org/?curid=164974). Tidal waves experience obstruction and deviation from the tide generating potential due to these factors. As a plane wave enters a basin, it is affected by the [Earth's rotation](https://en.wikipedia.org/?curid=4396171) and propagates along its borders, leading to the formation of [amphidromic points](https://en.wikipedia.org/?curid=336138) where tidal amplitude is zero and phase speed is infinite. The irregularity of oceanic basins and [bottom topography](https://en.wikipedia.org/?curid=164974) hinders precise mapping of tidal propagation, which was only made possible with the advent of satellite altimetry. Harmonic analysis of satellite altimetry data enables the generation of maps for different astronomical components. Amphidromic points and anti-[amphidromic points](https://en.wikipedia.org/?curid=336138), where tidal amplitude is maximum and phase is stationary, play a crucial role in understanding tidal behavior. The paper will further formalize these concepts to derive an expression for the tide generating potential. [ 78 ]
**The tide generating potential**
The section explains the calculation of tidal forces exerted by the Moon or Sun on the Earth's oceans. The tidal generating potential is analyzed in relation to the Earth's center, the tide generating body (TGB), and an observer at a point on the Earth's surface. The gravitational force exerted by the TGB is calculated, and the resulting force at the observer's point is determined. The potential is expressed in geographical coordinates, considering the observer's latitude and longitude, and the apparent latitude and longitude of the TGB. Spherical trigonometry is used to establish the relationship between the angles and positions of the TGB and the observer. The section also discusses the celestial reference point, Υ, and its movement with respect to the rotating Earth. The potential is then expressed in terms of the geographic coordinates and the apparent positions of the TGB, yielding the final tidal potential equation. [ 9 ]
**Pole**
The section discusses the Doodson constant, which is a coefficient related to the orbital distance of the tide generating body (TGB). The Doodson constant is used to calculate the long-period, diurnal, and semi-diurnal potential caused by the TGB. The paper presents plots of equipotential lines and cotidal/corange lines for the Earth tide, showing how the potential varies over time. It also mentions the variations in [declination](https://en.wikipedia.org/?curid=8612) and orbital distance and their impact on the potential. The response time of the Earth to the tidal potential is estimated to be much quicker than the tidal period, allowing the Earth to adjust to the equipotential surfaces. However, the oceans have a longer response time. The section sets the stage for considering how these variations affect the tidal potential in the subsequent sections of the paper. [ 11 ]
**Variation of the [declination](https://en.wikipedia.org/?curid=8612) of the tide generating body**
The research discusses the variation of the [declination](https://en.wikipedia.org/?curid=8612) of the tide generating body (TGB) in relation to its position relative to the equatorial plane. The paper explores the impact of the Earth's tilted rotational axis and the [precession](https://en.wikipedia.org/?curid=24714) of the equinoxes for the Sun, as well as the [precession](https://en.wikipedia.org/?curid=24714) of the lunar node for the Moon on the [declination](https://en.wikipedia.org/?curid=8612) of the Sun and Moon. It also addresses the variations in the orbital distance of the Moon and Sun from the Earth and their effect on tidal frequencies. The paper further investigates the modulation of basic tidal frequencies and provides a Fourier decomposition of the variations in [declination](https://en.wikipedia.org/?curid=8612) and distances to the TGBs. It concludes by presenting a spectrum for equilibrium tides, showcasing the cyclic patterns of tidal frequencies. [ 1417 ]
Tidal harmonics refer to the periodic components of the ocean tides, which are usually described using a harmonic analysis that breaks down the tidal signal into a series of sinusoidal components with specific frequencies and amplitudes. These components represent the various tidal constituents, each associated with gravitational forces from astronomical bodies such as the Moon, Sun, and other celestial factors, which collectively influence the complex tidal behavior observed in the world's oceans. By analyzing these harmonics, scientists can understand and predict tidal patterns and behavior in different locations.
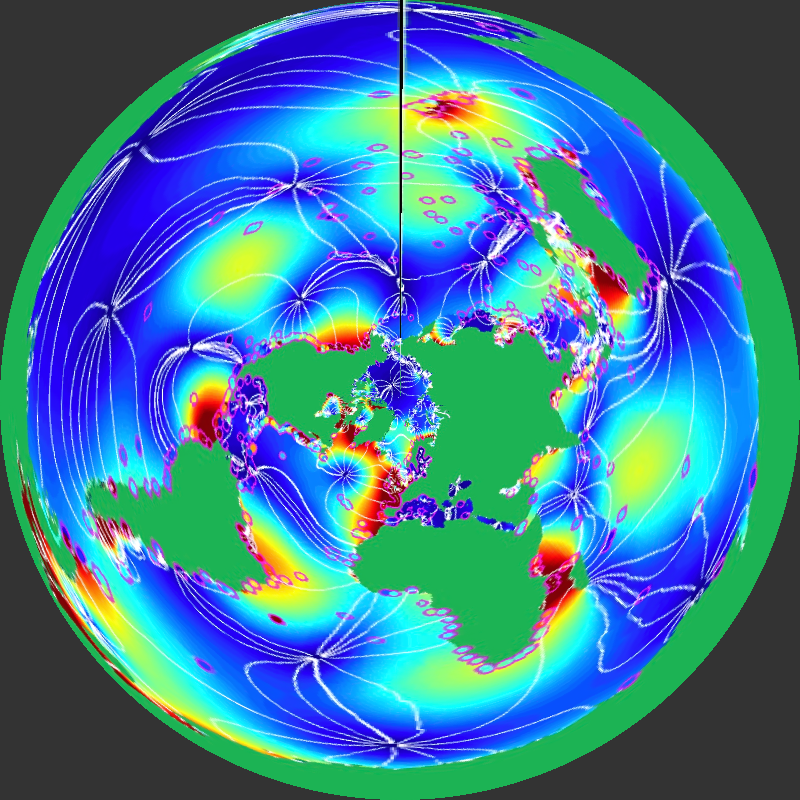
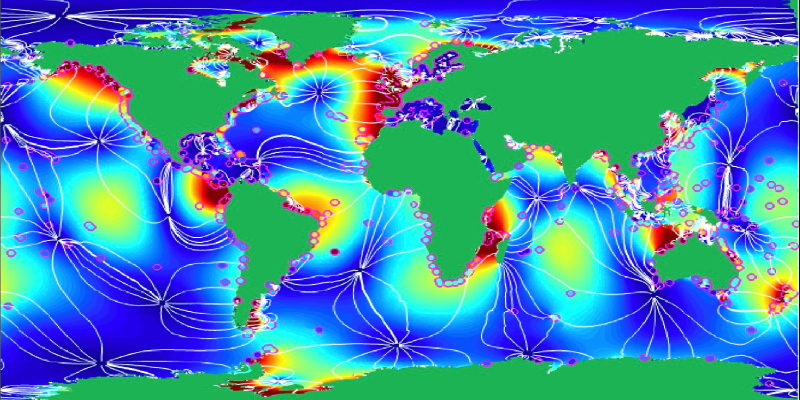
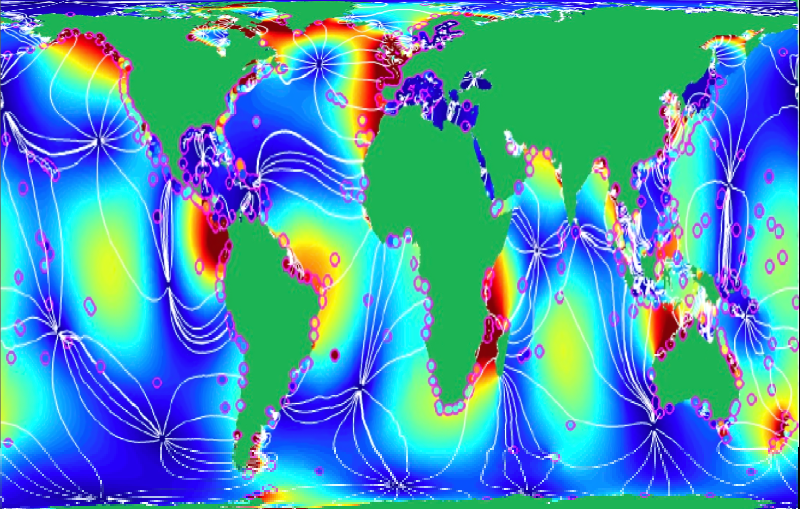
[https://www.canva.com/design/DAGBB6hbX2M/nAb1kt6BAmpQAuDyIhMVyQ/watch?utm_content=DAGBB6hbX2M&utm_campaign=designshare&utm_medium=link&utm_source=editor](https://www.canva.com/design/DAGBB6hbX2M/nAb1kt6BAmpQAuDyIhMVyQ/watch?utm_content=DAGBB6hbX2M&utm_campaign=designshare&utm_medium=link&utm_source=editor)
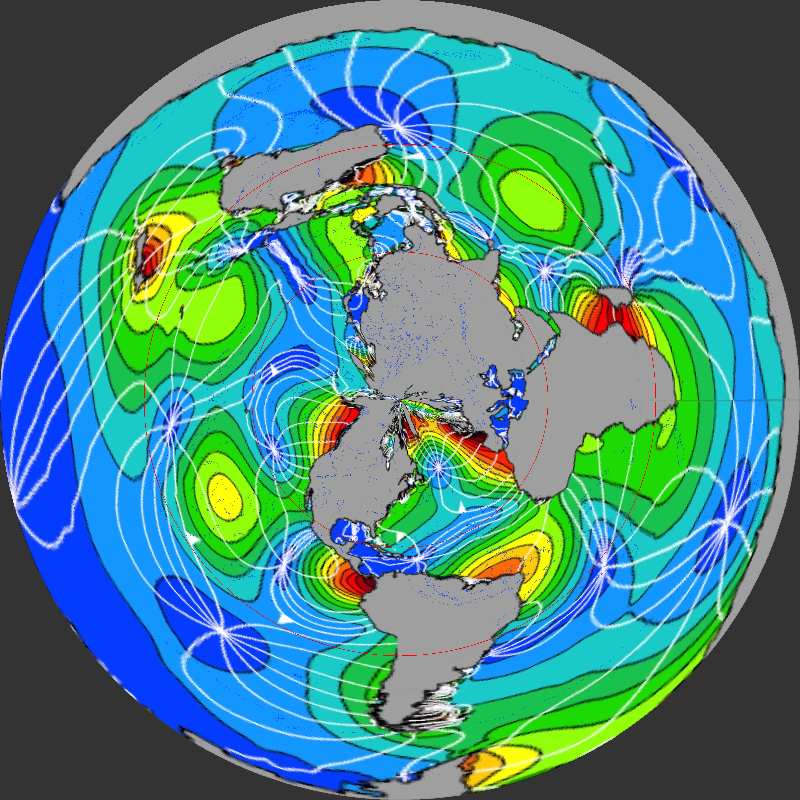
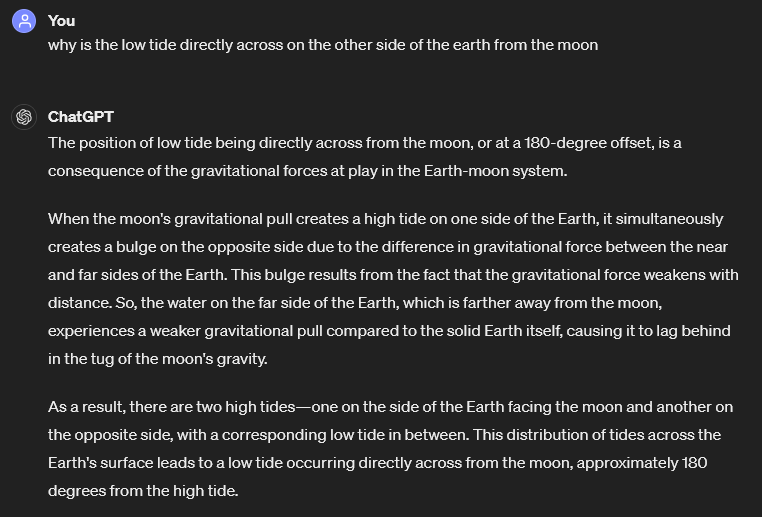
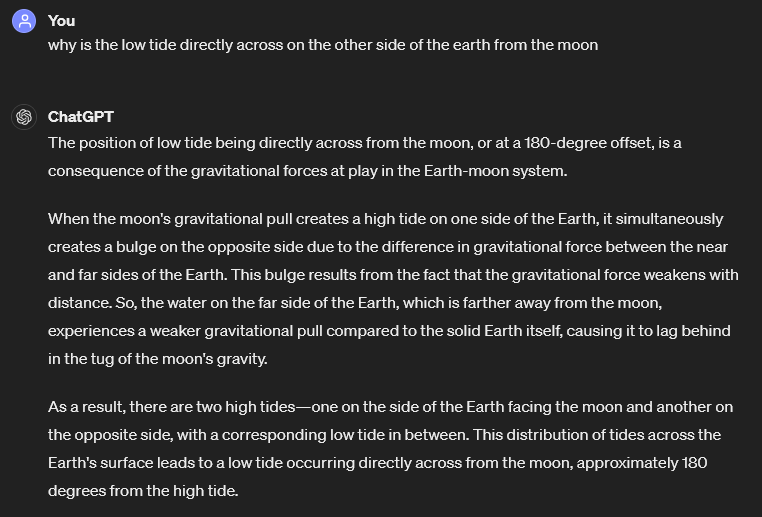
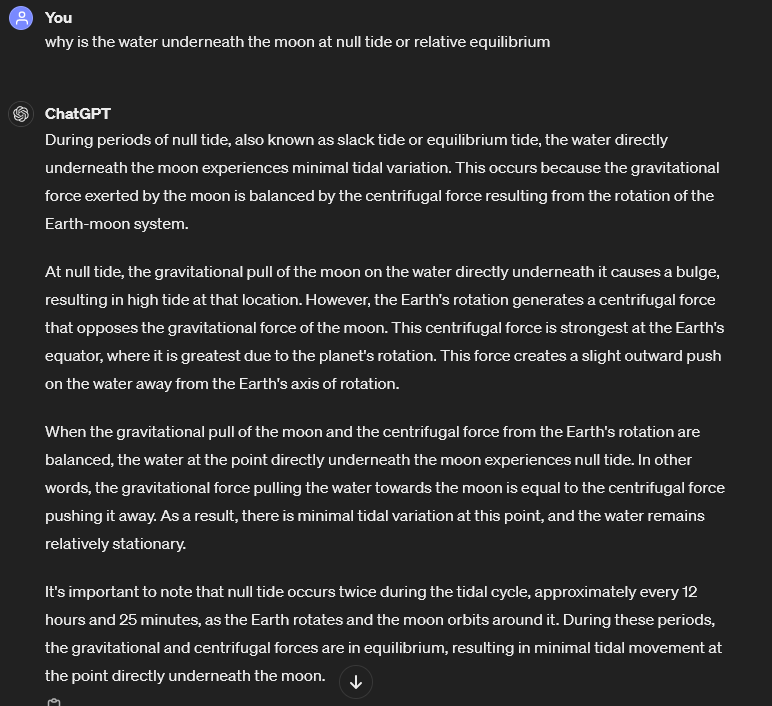
Links to this page
[Eclipses](https://publish.obsidian.md/shanesql/Eclipses)
[Geokinematics](https://publish.obsidian.md/shanesql/Geokinematics)